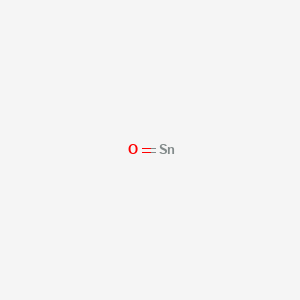
Tin(II) oxide
- Click on QUICK INQUIRY to receive a quote from our team of experts.
- With the quality product at a COMPETITIVE price, you can focus more on your research.
Overview
Description
Tin(II) oxide (SnO) is a metal oxide with wide applications in superconductivity, photovoltaics, optoelectronics as an anode, coating substrate, Schottky diode, and catalyst.
This compound appears as brownish-black powder or black to blue-black crystalline solid. Mp: 1080°C (decomposes); density: 6.45 g cm-3. Insoluble in water.
Scientific Research Applications
Gas Sensing Materials
Tin(II) oxide (SnO2) has significant applications as a solid-state gas sensor material. Its utility in this area is driven by its unique surface properties and electronic structure, influenced by the dual valency of Sn, which facilitates a reversible transformation of the surface composition. This property is critical in modifying the surface electronic structure and is central to the gas sensing mechanism of SnO2 (Batzill & Diebold, 2005).
Photoanodes in Dye-Sensitized Solar Cells
SnO2 is a candidate for applications requiring high electrical conductivity and optical transparency, such as photoanodes in dye-sensitized solar cells (DSSCs). It is chosen for its higher electron mobility and wider optical transparency compared to other metal oxide semiconductors. Despite some intrinsic limitations, recent improvements in SnO2-based DSSCs have shown promising results, including strategies to increase dye uptake and reduce recombination (Wali, Fakharuddin, & Jose, 2015).
Nanoparticle Synthesis
Tin oxide nanoparticles have received significant attention due to their improved properties when synthesized at the nanoscale. Green synthesis methods using biological entities like plant extracts and bacteria offer a cost-effective and environmentally friendly approach to producing tin oxide nanoparticles, although challenges remain in scaling these methods for industrial production (Gebreslassie & Gebretnsae, 2021).
Thin Films for Various Applications
This compound is also used in the deposition of thin films for various applications, such as in the creation of high-purity SnS films. The unique properties of SnO2 make it suitable for creating thin films with specific optoelectronic properties, crucial in applications like solar cells and chemical sensors (Ahmet et al., 2015).
Semiconductor and Catalytic Applications
As a wide band-gap n-type semiconductor, SnO2 has inherent potential for use as catalysts, sensors, and anode materials. Its structure-property relationship can be fine-tuned for specific functionalities, making it versatile for a range of applications (Mohanta & Ahmaruzzaman, 2016).
Mechanism of Action
Target of Action
Tin(II) oxide, also known as stannous oxide, is an inorganic compound composed of tin and oxygen where tin has the oxidation state of +2 . It primarily targets the oxidation-reduction processes in various chemical reactions .
Biochemical Pathways
This compound affects the oxidation-reduction pathways in biochemical reactions . It is thought to reduce copper(I) to metallic clusters in the manufacture of so-called "copper ruby glass" .
Pharmacokinetics
It is known that this compound is insoluble in water , which may impact its bioavailability.
Result of Action
The molecular and cellular effects of this compound’s action primarily involve changes in oxidation states. For instance, this compound can burn in air with a dim green flame to form SnO2 .
Action Environment
The action, efficacy, and stability of this compound are influenced by environmental factors such as temperature and atmospheric conditions. For example, at 300°C, the oxidation of this compound to Tin(IV) oxide proceeds incandescently . Furthermore, this compound is amphoteric, dissolving in strong acid to give tin(II) salts and in strong base to give stannites containing Sn(OH)3− .
Safety and Hazards
Future Directions
Tin(II) oxide has received tremendous attention due to its fascinating properties, which have been improved with the synthesis of this material in the nanometer range . It has potential applications in various fields such as energy storage, photocatalytic process, gas sensors, and solar cells . Future research will be addressed to evaluate the photocatalytic efficiency of tin oxide nanostructures to degrade organic contaminants in water .
Properties
{ "Design of the Synthesis Pathway": "The synthesis of Tin(II) oxide can be achieved through the reduction of Tin(IV) oxide using a reducing agent such as hydrogen gas or carbon monoxide gas.", "Starting Materials": [ "Tin(IV) oxide", "Hydrogen gas or Carbon monoxide gas" ], "Reaction": [ "Mix Tin(IV) oxide and the reducing agent in a reaction vessel", "Heat the reaction vessel to a temperature of 700-800°C", "Maintain the temperature for several hours to allow the reduction reaction to occur", "Cool the reaction vessel and collect the resulting Tin(II) oxide product" ] } | |
CAS No. |
21651-19-4 |
Molecular Formula |
OSn |
Molecular Weight |
134.71 g/mol |
IUPAC Name |
oxygen(2-);tin(2+) |
InChI |
InChI=1S/O.Sn/q-2;+2 |
InChI Key |
CJGYQECZUAUFSN-UHFFFAOYSA-N |
SMILES |
O=[Sn] |
Canonical SMILES |
[O-2].[Sn+2] |
boiling_point |
Decomposes (NIOSH, 2016) Decomposes |
density |
6.3 (NIOSH, 2016) 6.45 g/cm³ 6.3 |
melting_point |
1976 °F @ 600 mmHg. (Decomposes) (NIOSH, 2016) 1976°F@600 mmHg (decomposes) (600 mmHg): 1976°F (Decomposes) |
21651-19-4 | |
physical_description |
Tin(ii) oxide appears as brownish-black powder or black to blue-black crystalline solid. Mp: 1080°C (decomposes); density: 6.45 g cm-3. Insoluble in water. DryPowder; OtherSolid BLUE-TO-BLACK CRYSTALLINE POWDER. Brownish-black powder or black to blue-black crystalline solid. Brownish-black powder. |
Pictograms |
Irritant; Health Hazard |
solubility |
Insoluble (NIOSH, 2016) Solubility in water: none Insoluble |
vapor_pressure |
0 mm Hg (approx) (NIOSH, 2016) 0 mmHg 0 mmHg (approx) |
Origin of Product |
United States |
Disclaimer and Information on In-Vitro Research Products
Please be aware that all articles and product information presented on BenchChem are intended solely for informational purposes. The products available for purchase on BenchChem are specifically designed for in-vitro studies, which are conducted outside of living organisms. In-vitro studies, derived from the Latin term "in glass," involve experiments performed in controlled laboratory settings using cells or tissues. It is important to note that these products are not categorized as medicines or drugs, and they have not received approval from the FDA for the prevention, treatment, or cure of any medical condition, ailment, or disease. We must emphasize that any form of bodily introduction of these products into humans or animals is strictly prohibited by law. It is essential to adhere to these guidelines to ensure compliance with legal and ethical standards in research and experimentation.