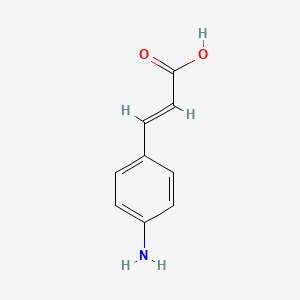
4-Aminocinnamic acid
Overview
Description
4-Aminocinnamic acid is an organic compound with the molecular formula C9H9NO2. It appears as a white crystalline solid and is soluble in hot water and ethanol, while being slightly soluble in ether and chloroform . This compound is known for its applications in organic synthesis and as a biochemical research probe.
Mechanism of Action
Target of Action
4-Aminocinnamic acid is primarily used in the modification of cellulose fibers, which are commonly used in medical and food packaging fields . The compound is chemically grafted onto dialdehyde cellulose fibers (DCFs) to yield antibacterial cellulose fibers .
Mode of Action
The exact mechanism of action of this compound isn’t completely understood. It’s postulated that the compound behaves as a nucleophile, interacting with electrophilic species such as carbonyl compounds and aldehydes . This interaction is thought to be responsible for the formation of cinnamates and amides, which are critical in the synthesis of various compounds .
Biochemical Pathways
This compound is involved in the modification of cellulose fibers through a Schiff base reaction . This process involves the chemical grafting of this compound onto dialdehyde cellulose fibers (DCFs), which are initially modified by sodium periodate (NaIO4) . The resulting this compound-modified DCFs exhibit excellent antibacterial activity .
Result of Action
The primary result of the action of this compound is the production of antibacterial cellulose fibers. The this compound-modified DCFs exhibit excellent antibacterial activity against S. aureus and E. coli, with inhibition ratios greater than 99.6% and 99.0%, respectively .
Action Environment
The action of this compound is influenced by environmental factors. For instance, the this compound-modified DCFs maintain a 99% antibacterial ratio after two months of exposure to the air environment . This suggests that the compound’s action, efficacy, and stability are robust against environmental changes.
Biochemical Analysis
Biochemical Properties
4-Aminocinnamic acid plays a significant role in biochemical reactions, particularly in the synthesis of antibacterial materials. It interacts with enzymes and proteins through Schiff base reactions, where the amino group of this compound forms a covalent bond with aldehyde groups on biomolecules . This interaction is crucial for the modification of cellulose fibers, endowing them with robust antibacterial properties against bacteria such as Staphylococcus aureus and Escherichia coli .
Cellular Effects
This compound exhibits notable effects on various cell types and cellular processes. It has been shown to influence cell function by modifying cellulose fibers, which then exhibit antibacterial activity. This modification leads to the inhibition of bacterial growth and colonization, thereby protecting the cells from microbial infections . Additionally, the compound’s interaction with cellular components can affect cell signaling pathways and gene expression, contributing to its antibacterial efficacy.
Molecular Mechanism
The molecular mechanism of this compound involves its ability to form Schiff bases with aldehyde groups on biomolecules. This reaction disrupts the bacterial cell membrane, leading to cell death . The compound’s antibacterial activity is primarily due to its ability to inhibit the growth of bacteria by interfering with their cellular processes. This mechanism highlights the potential of this compound as a valuable antibacterial agent.
Temporal Effects in Laboratory Settings
In laboratory settings, the effects of this compound have been observed to be stable over time. Studies have shown that cellulose fibers modified with this compound maintain their antibacterial properties for extended periods, with inhibition ratios remaining above 99% even after two months of exposure to the air environment . This stability makes this compound a promising candidate for long-term antibacterial applications.
Dosage Effects in Animal Models
The effects of this compound in animal models vary with different dosages. While specific dosage studies are limited, it is essential to consider the potential toxic or adverse effects at high doses. Understanding the threshold effects and optimal dosages is crucial for ensuring the safe and effective use of this compound in medical applications .
Metabolic Pathways
This compound is involved in metabolic pathways that include its interaction with enzymes such as phenylalanine ammonia-lyase (PAL). This enzyme catalyzes the conversion of phenylalanine to cinnamic acid, which is then further modified to form this compound . The compound’s involvement in these pathways highlights its role in the broader context of amino acid metabolism and its potential impact on metabolic flux and metabolite levels.
Transport and Distribution
Within cells and tissues, this compound is transported and distributed through interactions with specific transporters and binding proteins. These interactions influence the compound’s localization and accumulation, affecting its overall efficacy and function . Understanding these transport mechanisms is essential for optimizing the use of this compound in various applications.
Subcellular Localization
The subcellular localization of this compound is influenced by its chemical structure and interactions with cellular components. The compound is directed to specific compartments or organelles through targeting signals and post-translational modifications . This localization is crucial for its activity and function, particularly in the context of its antibacterial properties.
Preparation Methods
Synthetic Routes and Reaction Conditions: 4-Aminocinnamic acid can be synthesized through several methods. One common approach involves the reaction of benzaldehyde with ammonia in ethanol to form benzamide. This intermediate then undergoes a nucleophilic addition reaction with salicylaldehyde under basic conditions to yield this compound .
Industrial Production Methods: In industrial settings, this compound is often produced through the oxidation of cinnamaldehyde or by the condensation of benzal chloride with sodium acetate, followed by acid hydrolysis . These methods are preferred due to their efficiency and scalability.
Chemical Reactions Analysis
Types of Reactions: 4-Aminocinnamic acid undergoes various chemical reactions, including:
Oxidation: It can be oxidized to form corresponding quinones.
Reduction: It can be reduced to form 4-aminocinnamyl alcohol.
Substitution: It can participate in electrophilic aromatic substitution reactions.
Common Reagents and Conditions:
Oxidation: Potassium permanganate or chromium trioxide in acidic conditions.
Reduction: Sodium borohydride or lithium aluminum hydride.
Substitution: Halogens or nitro groups in the presence of a catalyst like iron or aluminum chloride.
Major Products:
Oxidation: Quinones.
Reduction: 4-Aminocinnamyl alcohol.
Substitution: Halogenated or nitrated derivatives of this compound.
Scientific Research Applications
4-Aminocinnamic acid has a wide range of applications in scientific research:
Chemistry: Used as a building block in organic synthesis.
Biology: Acts as a fluorescent probe in biochemical assays.
Medicine: Investigated for its potential antibacterial and anticancer properties.
Industry: Utilized in the production of antibacterial cellulose-based materials.
Comparison with Similar Compounds
Cinnamic Acid: Shares a similar structure but lacks the amino group.
4-Hydroxycinnamic Acid: Contains a hydroxyl group instead of an amino group.
3-Aminocinnamic Acid: The amino group is positioned differently on the aromatic ring.
Uniqueness: 4-Aminocinnamic acid is unique due to its amino group, which enhances its reactivity and allows for the formation of Schiff bases. This property is not present in cinnamic acid or 4-hydroxycinnamic acid, making this compound particularly valuable in synthetic and biological applications .
Properties
IUPAC Name |
(E)-3-(4-aminophenyl)prop-2-enoic acid | |
---|---|---|
Source | PubChem | |
URL | https://pubchem.ncbi.nlm.nih.gov | |
Description | Data deposited in or computed by PubChem | |
InChI |
InChI=1S/C9H9NO2/c10-8-4-1-7(2-5-8)3-6-9(11)12/h1-6H,10H2,(H,11,12)/b6-3+ | |
Source | PubChem | |
URL | https://pubchem.ncbi.nlm.nih.gov | |
Description | Data deposited in or computed by PubChem | |
InChI Key |
JOLPMPPNHIACPD-ZZXKWVIFSA-N | |
Source | PubChem | |
URL | https://pubchem.ncbi.nlm.nih.gov | |
Description | Data deposited in or computed by PubChem | |
Canonical SMILES |
C1=CC(=CC=C1C=CC(=O)O)N | |
Source | PubChem | |
URL | https://pubchem.ncbi.nlm.nih.gov | |
Description | Data deposited in or computed by PubChem | |
Isomeric SMILES |
C1=CC(=CC=C1/C=C/C(=O)O)N | |
Source | PubChem | |
URL | https://pubchem.ncbi.nlm.nih.gov | |
Description | Data deposited in or computed by PubChem | |
Molecular Formula |
C9H9NO2 | |
Source | PubChem | |
URL | https://pubchem.ncbi.nlm.nih.gov | |
Description | Data deposited in or computed by PubChem | |
DSSTOX Substance ID |
DTXSID40870966 | |
Record name | (2E)-3-(4-Aminophenyl)-2-propenoic acid | |
Source | EPA DSSTox | |
URL | https://comptox.epa.gov/dashboard/DTXSID40870966 | |
Description | DSSTox provides a high quality public chemistry resource for supporting improved predictive toxicology. | |
Molecular Weight |
163.17 g/mol | |
Source | PubChem | |
URL | https://pubchem.ncbi.nlm.nih.gov | |
Description | Data deposited in or computed by PubChem | |
CAS No. |
2393-18-2, 17570-30-8 | |
Record name | p-Aminocinnamic acid | |
Source | ChemIDplus | |
URL | https://pubchem.ncbi.nlm.nih.gov/substance/?source=chemidplus&sourceid=0002393182 | |
Description | ChemIDplus is a free, web search system that provides access to the structure and nomenclature authority files used for the identification of chemical substances cited in National Library of Medicine (NLM) databases, including the TOXNET system. | |
Record name | 2393-18-2 | |
Source | DTP/NCI | |
URL | https://dtp.cancer.gov/dtpstandard/servlet/dwindex?searchtype=NSC&outputformat=html&searchlist=1780 | |
Description | The NCI Development Therapeutics Program (DTP) provides services and resources to the academic and private-sector research communities worldwide to facilitate the discovery and development of new cancer therapeutic agents. | |
Explanation | Unless otherwise indicated, all text within NCI products is free of copyright and may be reused without our permission. Credit the National Cancer Institute as the source. | |
Record name | (2E)-3-(4-Aminophenyl)-2-propenoic acid | |
Source | EPA DSSTox | |
URL | https://comptox.epa.gov/dashboard/DTXSID40870966 | |
Description | DSSTox provides a high quality public chemistry resource for supporting improved predictive toxicology. | |
Record name | p-aminocinnamic acid | |
Source | European Chemicals Agency (ECHA) | |
URL | https://echa.europa.eu/substance-information/-/substanceinfo/100.017.498 | |
Description | The European Chemicals Agency (ECHA) is an agency of the European Union which is the driving force among regulatory authorities in implementing the EU's groundbreaking chemicals legislation for the benefit of human health and the environment as well as for innovation and competitiveness. | |
Explanation | Use of the information, documents and data from the ECHA website is subject to the terms and conditions of this Legal Notice, and subject to other binding limitations provided for under applicable law, the information, documents and data made available on the ECHA website may be reproduced, distributed and/or used, totally or in part, for non-commercial purposes provided that ECHA is acknowledged as the source: "Source: European Chemicals Agency, http://echa.europa.eu/". Such acknowledgement must be included in each copy of the material. ECHA permits and encourages organisations and individuals to create links to the ECHA website under the following cumulative conditions: Links can only be made to webpages that provide a link to the Legal Notice page. | |
Record name | 4-Aminocinnamic acid | |
Source | FDA Global Substance Registration System (GSRS) | |
URL | https://gsrs.ncats.nih.gov/ginas/app/beta/substances/DW9877G4G4 | |
Description | The FDA Global Substance Registration System (GSRS) enables the efficient and accurate exchange of information on what substances are in regulated products. Instead of relying on names, which vary across regulatory domains, countries, and regions, the GSRS knowledge base makes it possible for substances to be defined by standardized, scientific descriptions. | |
Explanation | Unless otherwise noted, the contents of the FDA website (www.fda.gov), both text and graphics, are not copyrighted. They are in the public domain and may be republished, reprinted and otherwise used freely by anyone without the need to obtain permission from FDA. Credit to the U.S. Food and Drug Administration as the source is appreciated but not required. | |
Synthesis routes and methods
Procedure details
Retrosynthesis Analysis
AI-Powered Synthesis Planning: Our tool employs the Template_relevance Pistachio, Template_relevance Bkms_metabolic, Template_relevance Pistachio_ringbreaker, Template_relevance Reaxys, Template_relevance Reaxys_biocatalysis model, leveraging a vast database of chemical reactions to predict feasible synthetic routes.
One-Step Synthesis Focus: Specifically designed for one-step synthesis, it provides concise and direct routes for your target compounds, streamlining the synthesis process.
Accurate Predictions: Utilizing the extensive PISTACHIO, BKMS_METABOLIC, PISTACHIO_RINGBREAKER, REAXYS, REAXYS_BIOCATALYSIS database, our tool offers high-accuracy predictions, reflecting the latest in chemical research and data.
Strategy Settings
Precursor scoring | Relevance Heuristic |
---|---|
Min. plausibility | 0.01 |
Model | Template_relevance |
Template Set | Pistachio/Bkms_metabolic/Pistachio_ringbreaker/Reaxys/Reaxys_biocatalysis |
Top-N result to add to graph | 6 |
Feasible Synthetic Routes
Disclaimer and Information on In-Vitro Research Products
Please be aware that all articles and product information presented on BenchChem are intended solely for informational purposes. The products available for purchase on BenchChem are specifically designed for in-vitro studies, which are conducted outside of living organisms. In-vitro studies, derived from the Latin term "in glass," involve experiments performed in controlled laboratory settings using cells or tissues. It is important to note that these products are not categorized as medicines or drugs, and they have not received approval from the FDA for the prevention, treatment, or cure of any medical condition, ailment, or disease. We must emphasize that any form of bodily introduction of these products into humans or animals is strictly prohibited by law. It is essential to adhere to these guidelines to ensure compliance with legal and ethical standards in research and experimentation.