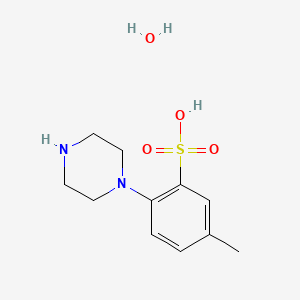
Caldaret monohydrate
Overview
Description
Preparation Methods
Synthetic Routes and Reaction Conditions: Caldaret monohydrate is synthesized through the condensation of 2-fluoro-5-methylbenzenesulfonic acid with piperazine in the presence of copper powder and cuprous iodide. This reaction is carried out in a sealed tube at 160°C. The resulting product is then converted to its more stable monohydrate form .
Industrial Production Methods: The industrial production of this compound involves similar synthetic routes but on a larger scale. The reaction conditions are optimized to ensure high yield and purity. The compound is then purified through crystallization and converted to its monohydrate form for stability and ease of handling .
Chemical Reactions Analysis
Types of Reactions: Caldaret monohydrate undergoes various chemical reactions, including:
Oxidation: It can be oxidized under specific conditions to form sulfonic acid derivatives.
Reduction: Reduction reactions can convert it to its corresponding amine derivatives.
Substitution: It can undergo nucleophilic substitution reactions, particularly at the sulfonic acid group.
Common Reagents and Conditions:
Oxidation: Common oxidizing agents include potassium permanganate and hydrogen peroxide.
Reduction: Reducing agents such as lithium aluminum hydride and sodium borohydride are used.
Substitution: Nucleophiles like amines and alcohols are commonly used in substitution reactions.
Major Products:
Oxidation: Sulfonic acid derivatives.
Reduction: Amine derivatives.
Substitution: Various substituted benzenesulfonic acid derivatives.
Scientific Research Applications
Caldaret monohydrate has a wide range of scientific research applications:
Chemistry: Used as a reagent in organic synthesis and as a model compound for studying sulfonic acid chemistry.
Biology: Investigated for its effects on intracellular calcium handling and its potential role in cellular signaling pathways.
Medicine: Primarily researched for its cardioprotective properties, particularly in reducing myocardial infarct size during reperfusion injury. .
Mechanism of Action
Caldaret monohydrate exerts its effects by modulating intracellular calcium handling. It inhibits the reverse mode of the sodium/calcium exchanger, leading to increased calcium uptake via the sarcoplasmic reticulum. This modulation helps in reducing calcium overload during ischemia and reperfusion, thereby protecting cardiac cells from damage .
Comparison with Similar Compounds
Diltiazem: Another calcium handling modulator used in the treatment of heart conditions.
Verapamil: A calcium channel blocker with similar cardioprotective properties.
Nifedipine: A dihydropyridine calcium channel blocker used for managing hypertension and angina.
Uniqueness of Caldaret Monohydrate: this compound is unique due to its specific mechanism of action involving the inhibition of the sodium/calcium exchanger and enhancement of sarcoplasmic reticulum calcium uptake. This dual action provides a more targeted approach to reducing myocardial infarct size and protecting cardiac cells during reperfusion injury .
Properties
CAS No. |
192509-24-3 |
---|---|
Molecular Formula |
C11H18N2O4S |
Molecular Weight |
274.34 g/mol |
IUPAC Name |
5-methyl-2-piperazin-1-ylbenzenesulfonic acid;hydrate |
InChI |
InChI=1S/C11H16N2O3S.H2O/c1-9-2-3-10(11(8-9)17(14,15)16)13-6-4-12-5-7-13;/h2-3,8,12H,4-7H2,1H3,(H,14,15,16);1H2 |
InChI Key |
ZRQKZWAOIRVBFD-UHFFFAOYSA-N |
SMILES |
CC1=CC(=C(C=C1)N2CCNCC2)S(=O)(=O)O.O |
Canonical SMILES |
CC1=CC(=C(C=C1)N2CCNCC2)S(=O)(=O)O.O |
192509-24-3 | |
Synonyms |
MCC 135 MCC-135 MCC135 |
Origin of Product |
United States |
Synthesis routes and methods I
Procedure details
Synthesis routes and methods II
Procedure details
Disclaimer and Information on In-Vitro Research Products
Please be aware that all articles and product information presented on BenchChem are intended solely for informational purposes. The products available for purchase on BenchChem are specifically designed for in-vitro studies, which are conducted outside of living organisms. In-vitro studies, derived from the Latin term "in glass," involve experiments performed in controlled laboratory settings using cells or tissues. It is important to note that these products are not categorized as medicines or drugs, and they have not received approval from the FDA for the prevention, treatment, or cure of any medical condition, ailment, or disease. We must emphasize that any form of bodily introduction of these products into humans or animals is strictly prohibited by law. It is essential to adhere to these guidelines to ensure compliance with legal and ethical standards in research and experimentation.