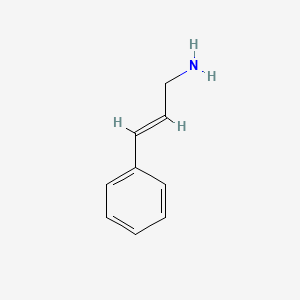
Cinnamylamine
Overview
Description
Cinnamylamine is an aromatic compound derived from l-phenylalanine. It is used in the synthesis of biologically active molecules, including drugs and energetic materials. This compound is valuable for its antibacterial, antiviral, and anticancer properties, making it a significant starting material in various chemical syntheses .
Mechanism of Action
Target of Action
Cinnamylamine is an aromatic compound derived from L-phenylalanine . The primary target of this compound is the ω-aminotransferase Cv-ωTA from Chromobacterium violaceum . This enzyme plays a crucial role in the conversion of cinnamaldehyde to this compound .
Mode of Action
The Cv-ωTA enzyme exhibits high activity in the conversion of cinnamaldehyde to this compound . This conversion is a key step in the biosynthesis of this compound . To shift the equilibrium of the reaction towards this compound, saturation mutagenesis of Cv-ωTA at key amino acid residues was performed .
Biochemical Pathways
The biosynthesis of this compound involves several steps . First, the carboxylic acid reductase from Neurospora crassa (NcCAR) and phosphopantetheinyl transferase (PPTase) from E. coli are used to convert cinnamic acid to cinnamaldehyde . Then, the Cv-ωTA enzyme converts cinnamaldehyde to this compound . This process is part of a larger metabolic pathway involving the conversion of L-phenylalanine to cinnamic acid .
Pharmacokinetics
coli has been optimized by adjusting the supply of the cofactors, PLP and NADPH, in the fermentation process . This suggests that these factors may play a role in the bioavailability of this compound.
Result of Action
The result of the action of this compound is the production of an aromatic compound that can be used in the synthesis of biologically active molecules, including drugs, and energetic materials . The highest reported yield of this compound in engineered E. coli reached 523.15 mg/L .
Action Environment
The action of this compound can be influenced by various environmental factors. For instance, the yield of this compound in engineered E. coli was improved by optimizing the substrates and the supply of the cofactors, PLP and NADPH, in the fermentation process . Additionally, the overexpression or knockout of certain native global transcription factors and resistance genes in E. coli was found to regulate the catalytic rate of NcCAR and Cv-ωTA, thereby affecting the production of this compound .
Biochemical Analysis
Biochemical Properties
Cinnamylamine plays a significant role in biochemical reactions, particularly in the synthesis of aromatic primary amines. It interacts with enzymes such as carboxylic acid reductase from Neurospora crassa and ω-transaminase from Ochrobactrum anthropi. These enzymes catalyze the conversion of cinnamic acid to this compound through a series of biocatalytic reactions. The interaction between this compound and these enzymes is crucial for the efficient production of the compound .
Cellular Effects
This compound influences various cellular processes, including cell signaling pathways, gene expression, and cellular metabolism. In Escherichia coli, the overexpression or knockout of specific transcription factors and resistance genes can regulate the production of this compound. This regulation affects the fluxes of NADPH and ATP, as well as the rate of pyruvate metabolism. Additionally, this compound can upregulate genes related to stress and detoxification, impacting overall cellular function .
Molecular Mechanism
At the molecular level, this compound exerts its effects through binding interactions with specific enzymes and proteins. The compound is involved in enzyme inhibition and activation, particularly in the catalytic processes of carboxylic acid reductase and ω-transaminase. These interactions lead to changes in gene expression and metabolic pathways, ultimately influencing the synthesis and stability of this compound .
Temporal Effects in Laboratory Settings
In laboratory settings, the effects of this compound can change over time due to its stability and degradation. Studies have shown that the accumulation of toxic intermediates, such as aromatic aldehydes, can affect the yield of this compound. Metabolic regulation through the overexpression or knockout of specific genes can enhance the stability and production of this compound over time .
Dosage Effects in Animal Models
The effects of this compound vary with different dosages in animal models. At lower doses, the compound may exhibit beneficial effects, such as antifungal and analgesic properties. At higher doses, this compound can cause toxic or adverse effects, including metabolic imbalances and cellular stress. It is essential to determine the optimal dosage to maximize the therapeutic benefits while minimizing potential risks .
Metabolic Pathways
This compound is involved in several metabolic pathways, including the shikimate pathway and the phenylalanine lyase pathway. These pathways facilitate the conversion of cinnamic acid to this compound through a series of enzymatic reactions. Enzymes such as carboxylic acid reductase and ω-transaminase play a crucial role in these pathways, influencing the metabolic flux and levels of metabolites .
Transport and Distribution
Within cells and tissues, this compound is transported and distributed through specific transporters and binding proteins. These interactions affect the localization and accumulation of the compound, influencing its overall activity and function. The transport and distribution of this compound are essential for its effective utilization in biochemical reactions and therapeutic applications .
Subcellular Localization
This compound’s subcellular localization is influenced by targeting signals and post-translational modifications. These factors direct the compound to specific compartments or organelles within the cell, affecting its activity and function. Understanding the subcellular localization of this compound is crucial for optimizing its therapeutic potential and minimizing potential side effects .
Preparation Methods
Synthetic Routes and Reaction Conditions: Cinnamylamine can be synthesized through chemical methods, primarily involving the conversion of cinnamaldehyde to this compound using ω-aminotransferase from Chromobacterium violaceum. This process can be optimized by employing engineered Escherichia coli strains to enhance the conversion rate .
Industrial Production Methods: Industrial production of this compound involves biocatalytic synthesis using engineered strains of Escherichia coli. The process includes the use of carboxylic acid reductase from Neurospora crassa and phosphopantetheinyl transferase from Escherichia coli to convert cinnamic acid to cinnamaldehyde, followed by its conversion to this compound .
Chemical Reactions Analysis
Types of Reactions: Cinnamylamine undergoes various chemical reactions, including oxidation, reduction, and substitution.
Common Reagents and Conditions:
Oxidation: Typically involves reagents like potassium permanganate or chromium trioxide.
Reduction: Commonly uses hydrogen gas in the presence of a palladium catalyst.
Substitution: Often involves halogenation reactions using reagents like bromine or chlorine.
Major Products: The major products formed from these reactions include cinnamaldehyde, cinnamyl alcohol, and various substituted cinnamylamines .
Scientific Research Applications
Cinnamylamine has extensive applications in scientific research:
Chemistry: Used as a precursor for synthesizing bioactive substances such as pyrazines and quinazolinones.
Biology: Plays a role in the synthesis of compounds with antibacterial, antiviral, and anticancer properties.
Medicine: Utilized in the development of drugs for treating infections and cancer.
Industry: Serves as a precursor for energetic materials and other industrial chemicals
Comparison with Similar Compounds
4-Fluorobenzylamine: An intermediate in the synthesis of analgesics.
2-Furfurylamine: Used in the production of diuretics.
2,4-Difluorobenzylamine: A key intermediate in the synthesis of anti-HIV drugs.
Uniqueness: Cinnamylamine is unique due to its aromatic structure and the presence of an amine group, which makes it a versatile precursor for synthesizing a wide range of bioactive compounds. Its ability to undergo various chemical reactions and its extensive applications in different fields highlight its significance .
Biological Activity
Cinnamylamine, an aromatic amine derived from cinnamic acid, has attracted significant attention in recent years due to its potential applications in medicinal chemistry and biocatalytic processes. This article explores the biological activity of this compound, focusing on its synthesis, metabolic engineering, and potential therapeutic uses.
Chemical Structure and Properties
This compound is characterized by its structure, which includes a phenyl group attached to an amino group via a propenyl chain. This configuration contributes to its reactivity and biological activity. The compound can be represented as follows:
Synthesis of this compound
Traditionally, this compound has been synthesized through chemical methods; however, recent advances in metabolic engineering have facilitated its biosynthesis using microbial systems. Key studies have focused on optimizing the production of this compound via engineered Escherichia coli strains.
Biosynthetic Pathways
- Enzymatic Conversion : The ω-aminotransferase from Chromobacterium violaceum (Cv-ωTA) has shown high efficiency in converting cinnamaldehyde to this compound. This enzymatic pathway is pivotal for enhancing the yield of this compound during fermentation processes .
-
Metabolic Engineering : Researchers have employed various strategies to optimize the biosynthesis route:
- Gene Knockout : Knocking out specific genes in E. coli that encode aldehyde reductases and alcohol dehydrogenases prevents unwanted side reactions, thus favoring the conversion to this compound .
- Co-factor Optimization : The supply of pyridoxal phosphate (PLP) and NADPH is critical for maximizing the yield during fermentation processes .
Yield Optimization Studies
Recent studies have reported significant advancements in the yield of this compound through metabolic engineering:
Biological Activity and Therapeutic Potential
This compound exhibits various biological activities that make it a candidate for pharmaceutical applications:
- Antimicrobial Properties : Preliminary studies suggest that this compound may possess antimicrobial effects, making it a potential agent for treating infections .
- Pharmacophore in Medicinal Chemistry : Cinnamylamines and their derivatives are recognized as important pharmacophores in drug development, particularly in the synthesis of compounds with anti-inflammatory and analgesic properties .
Case Studies
- Synergistic Production Enhancement : A study demonstrated that by metabolically regulating transcription factors in E. coli, researchers could enhance the production of this compound significantly, achieving yields comparable to traditional chemical methods .
- Biosynthesis Reference Framework : Another study established a foundational biosynthetic route for this compound, paving the way for future research into similar aromatic amines .
Properties
IUPAC Name |
(E)-3-phenylprop-2-en-1-amine | |
---|---|---|
Source | PubChem | |
URL | https://pubchem.ncbi.nlm.nih.gov | |
Description | Data deposited in or computed by PubChem | |
InChI |
InChI=1S/C9H11N/c10-8-4-7-9-5-2-1-3-6-9/h1-7H,8,10H2/b7-4+ | |
Source | PubChem | |
URL | https://pubchem.ncbi.nlm.nih.gov | |
Description | Data deposited in or computed by PubChem | |
InChI Key |
RDAFNSMYPSHCBK-QPJJXVBHSA-N | |
Source | PubChem | |
URL | https://pubchem.ncbi.nlm.nih.gov | |
Description | Data deposited in or computed by PubChem | |
Canonical SMILES |
C1=CC=C(C=C1)C=CCN | |
Source | PubChem | |
URL | https://pubchem.ncbi.nlm.nih.gov | |
Description | Data deposited in or computed by PubChem | |
Isomeric SMILES |
C1=CC=C(C=C1)/C=C/CN | |
Source | PubChem | |
URL | https://pubchem.ncbi.nlm.nih.gov | |
Description | Data deposited in or computed by PubChem | |
Molecular Formula |
C9H11N | |
Source | PubChem | |
URL | https://pubchem.ncbi.nlm.nih.gov | |
Description | Data deposited in or computed by PubChem | |
Molecular Weight |
133.19 g/mol | |
Source | PubChem | |
URL | https://pubchem.ncbi.nlm.nih.gov | |
Description | Data deposited in or computed by PubChem | |
CAS No. |
4335-60-8, 4360-51-4 | |
Record name | Cinnamylamine, (E)- | |
Source | ChemIDplus | |
URL | https://pubchem.ncbi.nlm.nih.gov/substance/?source=chemidplus&sourceid=0004335608 | |
Description | ChemIDplus is a free, web search system that provides access to the structure and nomenclature authority files used for the identification of chemical substances cited in National Library of Medicine (NLM) databases, including the TOXNET system. | |
Record name | Cinnamylamine | |
Source | ChemIDplus | |
URL | https://pubchem.ncbi.nlm.nih.gov/substance/?source=chemidplus&sourceid=0004360514 | |
Description | ChemIDplus is a free, web search system that provides access to the structure and nomenclature authority files used for the identification of chemical substances cited in National Library of Medicine (NLM) databases, including the TOXNET system. | |
Record name | CINNAMYLAMINE, (E)- | |
Source | FDA Global Substance Registration System (GSRS) | |
URL | https://gsrs.ncats.nih.gov/ginas/app/beta/substances/KRU5188QAR | |
Description | The FDA Global Substance Registration System (GSRS) enables the efficient and accurate exchange of information on what substances are in regulated products. Instead of relying on names, which vary across regulatory domains, countries, and regions, the GSRS knowledge base makes it possible for substances to be defined by standardized, scientific descriptions. | |
Explanation | Unless otherwise noted, the contents of the FDA website (www.fda.gov), both text and graphics, are not copyrighted. They are in the public domain and may be republished, reprinted and otherwise used freely by anyone without the need to obtain permission from FDA. Credit to the U.S. Food and Drug Administration as the source is appreciated but not required. | |
Synthesis routes and methods I
Procedure details
Synthesis routes and methods II
Procedure details
Retrosynthesis Analysis
AI-Powered Synthesis Planning: Our tool employs the Template_relevance Pistachio, Template_relevance Bkms_metabolic, Template_relevance Pistachio_ringbreaker, Template_relevance Reaxys, Template_relevance Reaxys_biocatalysis model, leveraging a vast database of chemical reactions to predict feasible synthetic routes.
One-Step Synthesis Focus: Specifically designed for one-step synthesis, it provides concise and direct routes for your target compounds, streamlining the synthesis process.
Accurate Predictions: Utilizing the extensive PISTACHIO, BKMS_METABOLIC, PISTACHIO_RINGBREAKER, REAXYS, REAXYS_BIOCATALYSIS database, our tool offers high-accuracy predictions, reflecting the latest in chemical research and data.
Strategy Settings
Precursor scoring | Relevance Heuristic |
---|---|
Min. plausibility | 0.01 |
Model | Template_relevance |
Template Set | Pistachio/Bkms_metabolic/Pistachio_ringbreaker/Reaxys/Reaxys_biocatalysis |
Top-N result to add to graph | 6 |
Feasible Synthetic Routes
Disclaimer and Information on In-Vitro Research Products
Please be aware that all articles and product information presented on BenchChem are intended solely for informational purposes. The products available for purchase on BenchChem are specifically designed for in-vitro studies, which are conducted outside of living organisms. In-vitro studies, derived from the Latin term "in glass," involve experiments performed in controlled laboratory settings using cells or tissues. It is important to note that these products are not categorized as medicines or drugs, and they have not received approval from the FDA for the prevention, treatment, or cure of any medical condition, ailment, or disease. We must emphasize that any form of bodily introduction of these products into humans or animals is strictly prohibited by law. It is essential to adhere to these guidelines to ensure compliance with legal and ethical standards in research and experimentation.