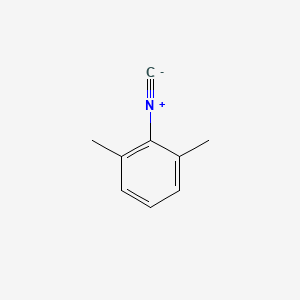
2,6-Dimethylphenyl isocyanide
Overview
Description
2,6-Dimethylphenyl isocyanide, also known as vicinal-meta-xylyl isocyanide, is an organic compound with the molecular formula C₉H₉N. It is a colorless liquid with a strong, pungent odor characteristic of isocyanides. This compound is used in various organic synthesis processes and has applications in the preparation of complex molecules .
Scientific Research Applications
2,6-Dimethylphenyl isocyanide has several applications in scientific research:
Organic Synthesis: Used in the synthesis of complex organic molecules, including metallaamidine complexes and derivatized cyclodextrins.
Chiral Stationary Phases: Employed in normal-phase liquid chromatography as a chiral stationary phase.
Biological Studies: Investigated for its binding properties to proteins such as myoglobin and hemoglobin.
Safety and Hazards
When handling 2,6-Dimethylphenyl isocyanide, it is recommended to wash face, hands, and any exposed skin thoroughly. It is advised not to eat, drink, or smoke when using this product. Protective gloves, clothing, eye protection, and face protection should be worn. It is also advised not to breathe dust, fume, gas, mist, vapors, or spray. This product should be used only outdoors or in a well-ventilated area. Respiratory protection should be worn .
Mechanism of Action
Target of Action
2,6-Dimethylphenyl isocyanide (Dmp-NC) is a versatile compound that interacts with various targets. It is often used as a ligand in the synthesis of transition metal catalysts . The primary targets of Dmp-NC are the metal centers of these catalysts, where it binds and influences their reactivity .
Mode of Action
Dmp-NC interacts with its targets through coordination to the metal centers of catalysts . In the presence of these catalysts, Dmp-NC can undergo various transformations. For instance, it can participate in controlled reductive C–C coupling reactions promoted by an aluminyl anion . This reaction leads to the formation of a C3-homologation product, involving C–C bond formation and dearomatisation of one of the aromatic substituents .
Biochemical Pathways
The biochemical pathways affected by Dmp-NC primarily involve the synthesis of nitrogen-containing organic molecules . Dmp-NC can also undergo (4+1) cycloaddition reaction with certain α,β-unsaturated ketones in the presence of a GaCl3 catalyst to give the corresponding unsaturated lactones .
Result of Action
The action of Dmp-NC results in the formation of new organic compounds. For example, its reaction with an aluminyl anion leads to the formation of a C3-homologation product . Additionally, its reaction with α,β-unsaturated ketones results in the formation of unsaturated lactones .
Action Environment
The action, efficacy, and stability of Dmp-NC are highly dependent on the environmental conditions, including the presence of other reactants, the type of catalyst used, and the specific reaction conditions . For instance, the use of an aluminyl anion as a catalyst promotes the reductive C–C coupling of Dmp-NC , while the use of a GaCl3 catalyst facilitates its cycloaddition reaction with α,β-unsaturated ketones .
Biochemical Analysis
Biochemical Properties
2,6-Dimethylphenyl isocyanide plays a significant role in biochemical reactions, particularly in the synthesis of complex organic molecules. It interacts with various enzymes, proteins, and other biomolecules. For instance, it has been found to bind to sheep hemoglobin and horse heart myoglobin with high affinity
Cellular Effects
The effects of this compound on various types of cells and cellular processes are profound. It influences cell function by affecting cell signaling pathways, gene expression, and cellular metabolism. Studies have shown that this compound can inhibit the activity of certain enzymes involved in cellular metabolism, leading to altered metabolic flux and changes in metabolite levels . This compound’s impact on gene expression and cell signaling pathways further underscores its potential as a biochemical tool in research.
Molecular Mechanism
At the molecular level, this compound exerts its effects through specific binding interactions with biomolecules. It has been shown to inhibit the activity of enzymes such as FabF and GlmS by covalently binding to their active site cysteines . This inhibition disrupts essential metabolic pathways, leading to significant changes in cellular function. Additionally, this compound can induce changes in gene expression, further influencing cellular processes.
Temporal Effects in Laboratory Settings
The stability and degradation of this compound in laboratory settings are critical factors in its application. Over time, the compound may undergo degradation, affecting its efficacy and potency. Long-term studies have shown that this compound can have lasting effects on cellular function, with some changes persisting even after the compound has been metabolized . Understanding these temporal effects is essential for optimizing its use in biochemical research.
Dosage Effects in Animal Models
The effects of this compound vary with different dosages in animal models. At low doses, the compound may exhibit minimal toxicity and significant biochemical activity. At higher doses, it can cause adverse effects, including respiratory and dermal sensitization . These dosage-dependent effects highlight the importance of careful dosage optimization in experimental settings to balance efficacy and safety.
Metabolic Pathways
This compound is involved in several metabolic pathways, interacting with various enzymes and cofactors. It has been shown to affect the fatty acid biosynthetic process and the hexosamine pathway by covalently modifying key enzymes . These interactions can lead to changes in metabolic flux and alterations in metabolite levels, providing insights into the compound’s role in cellular metabolism.
Transport and Distribution
The transport and distribution of this compound within cells and tissues are influenced by its interactions with transporters and binding proteins. The compound’s localization and accumulation in specific cellular compartments can affect its activity and function . Understanding these transport mechanisms is crucial for predicting the compound’s behavior in biological systems.
Subcellular Localization
This compound’s subcellular localization is determined by targeting signals and post-translational modifications that direct it to specific compartments or organelles. These localization patterns can influence the compound’s activity and function, providing insights into its role in cellular processes
Preparation Methods
Synthetic Routes and Reaction Conditions
2,6-Dimethylphenyl isocyanide can be synthesized through several methods. One common method involves the reaction of 2,6-dimethylaniline with chloroform and potassium hydroxide in the presence of a phase-transfer catalyst. The reaction proceeds via the formation of an intermediate isocyanide, which is then isolated and purified .
Industrial Production Methods
In industrial settings, the production of this compound typically involves large-scale reactions using similar reagents and conditions as in laboratory synthesis. The process is optimized for higher yields and purity, often employing continuous flow reactors and advanced purification techniques .
Chemical Reactions Analysis
Types of Reactions
2,6-Dimethylphenyl isocyanide undergoes various chemical reactions, including:
Cycloaddition Reactions: It can participate in (4+1) cycloaddition reactions with α,β-unsaturated ketones in the presence of a gallium trichloride catalyst to form unsaturated lactones.
Insertion Reactions: It can insert into metal-silyl bonds, such as in the reaction with zirconium-silyl complexes.
Common Reagents and Conditions
Gallium Trichloride: Used as a catalyst in cycloaddition reactions.
Triphenylphosphine: Catalyzes the reaction with diphenylcyclopropenone to form N-(2,6-dimethylphenyl)diphenyliminocyclobutenone.
Major Products
Unsaturated Lactones: Formed from cycloaddition reactions.
N-(2,6-Dimethylphenyl)diphenyliminocyclobutenone: Formed from the reaction with diphenylcyclopropenone.
Comparison with Similar Compounds
Similar Compounds
2,6-Dimethylphenyl isocyanate: Similar in structure but contains an isocyanate group instead of an isocyanide group.
2,6-Dimethylaniline: The precursor in the synthesis of 2,6-dimethylphenyl isocyanide.
Benzyl isocyanide: Another isocyanide compound with different substituents on the aromatic ring.
Uniqueness
This compound is unique due to its specific substitution pattern on the aromatic ring, which influences its reactivity and binding properties. This makes it particularly useful in the synthesis of specialized organic compounds and in applications requiring specific ligand properties .
Properties
IUPAC Name |
2-isocyano-1,3-dimethylbenzene | |
---|---|---|
Source | PubChem | |
URL | https://pubchem.ncbi.nlm.nih.gov | |
Description | Data deposited in or computed by PubChem | |
InChI |
InChI=1S/C9H9N/c1-7-5-4-6-8(2)9(7)10-3/h4-6H,1-2H3 | |
Source | PubChem | |
URL | https://pubchem.ncbi.nlm.nih.gov | |
Description | Data deposited in or computed by PubChem | |
InChI Key |
DNJLFZHMJDSJFN-UHFFFAOYSA-N | |
Source | PubChem | |
URL | https://pubchem.ncbi.nlm.nih.gov | |
Description | Data deposited in or computed by PubChem | |
Canonical SMILES |
CC1=C(C(=CC=C1)C)[N+]#[C-] | |
Source | PubChem | |
URL | https://pubchem.ncbi.nlm.nih.gov | |
Description | Data deposited in or computed by PubChem | |
Molecular Formula |
C9H9N | |
Source | PubChem | |
URL | https://pubchem.ncbi.nlm.nih.gov | |
Description | Data deposited in or computed by PubChem | |
DSSTOX Substance ID |
DTXSID10182048 | |
Record name | 2,6-Dimethylphenylisonitrile | |
Source | EPA DSSTox | |
URL | https://comptox.epa.gov/dashboard/DTXSID10182048 | |
Description | DSSTox provides a high quality public chemistry resource for supporting improved predictive toxicology. | |
Molecular Weight |
131.17 g/mol | |
Source | PubChem | |
URL | https://pubchem.ncbi.nlm.nih.gov | |
Description | Data deposited in or computed by PubChem | |
CAS No. |
2769-71-3 | |
Record name | 2,6-Dimethylphenyl isocyanide | |
Source | CAS Common Chemistry | |
URL | https://commonchemistry.cas.org/detail?cas_rn=2769-71-3 | |
Description | CAS Common Chemistry is an open community resource for accessing chemical information. Nearly 500,000 chemical substances from CAS REGISTRY cover areas of community interest, including common and frequently regulated chemicals, and those relevant to high school and undergraduate chemistry classes. This chemical information, curated by our expert scientists, is provided in alignment with our mission as a division of the American Chemical Society. | |
Explanation | The data from CAS Common Chemistry is provided under a CC-BY-NC 4.0 license, unless otherwise stated. | |
Record name | 2,6-Dimethylphenyl isocyanide | |
Source | ChemIDplus | |
URL | https://pubchem.ncbi.nlm.nih.gov/substance/?source=chemidplus&sourceid=0002769713 | |
Description | ChemIDplus is a free, web search system that provides access to the structure and nomenclature authority files used for the identification of chemical substances cited in National Library of Medicine (NLM) databases, including the TOXNET system. | |
Record name | 2,6-Dimethylphenyl isocyanide | |
Source | DTP/NCI | |
URL | https://dtp.cancer.gov/dtpstandard/servlet/dwindex?searchtype=NSC&outputformat=html&searchlist=141690 | |
Description | The NCI Development Therapeutics Program (DTP) provides services and resources to the academic and private-sector research communities worldwide to facilitate the discovery and development of new cancer therapeutic agents. | |
Explanation | Unless otherwise indicated, all text within NCI products is free of copyright and may be reused without our permission. Credit the National Cancer Institute as the source. | |
Record name | 2,6-Dimethylphenylisonitrile | |
Source | EPA DSSTox | |
URL | https://comptox.epa.gov/dashboard/DTXSID10182048 | |
Description | DSSTox provides a high quality public chemistry resource for supporting improved predictive toxicology. | |
Record name | 2,6-xylyl isocyanide | |
Source | European Chemicals Agency (ECHA) | |
URL | https://echa.europa.eu/substance-information/-/substanceinfo/100.018.600 | |
Description | The European Chemicals Agency (ECHA) is an agency of the European Union which is the driving force among regulatory authorities in implementing the EU's groundbreaking chemicals legislation for the benefit of human health and the environment as well as for innovation and competitiveness. | |
Explanation | Use of the information, documents and data from the ECHA website is subject to the terms and conditions of this Legal Notice, and subject to other binding limitations provided for under applicable law, the information, documents and data made available on the ECHA website may be reproduced, distributed and/or used, totally or in part, for non-commercial purposes provided that ECHA is acknowledged as the source: "Source: European Chemicals Agency, http://echa.europa.eu/". Such acknowledgement must be included in each copy of the material. ECHA permits and encourages organisations and individuals to create links to the ECHA website under the following cumulative conditions: Links can only be made to webpages that provide a link to the Legal Notice page. | |
Record name | 2,6-XYLYL ISONITRILE | |
Source | FDA Global Substance Registration System (GSRS) | |
URL | https://gsrs.ncats.nih.gov/ginas/app/beta/substances/TD977ELU5R | |
Description | The FDA Global Substance Registration System (GSRS) enables the efficient and accurate exchange of information on what substances are in regulated products. Instead of relying on names, which vary across regulatory domains, countries, and regions, the GSRS knowledge base makes it possible for substances to be defined by standardized, scientific descriptions. | |
Explanation | Unless otherwise noted, the contents of the FDA website (www.fda.gov), both text and graphics, are not copyrighted. They are in the public domain and may be republished, reprinted and otherwise used freely by anyone without the need to obtain permission from FDA. Credit to the U.S. Food and Drug Administration as the source is appreciated but not required. | |
Synthesis routes and methods
Procedure details
Retrosynthesis Analysis
AI-Powered Synthesis Planning: Our tool employs the Template_relevance Pistachio, Template_relevance Bkms_metabolic, Template_relevance Pistachio_ringbreaker, Template_relevance Reaxys, Template_relevance Reaxys_biocatalysis model, leveraging a vast database of chemical reactions to predict feasible synthetic routes.
One-Step Synthesis Focus: Specifically designed for one-step synthesis, it provides concise and direct routes for your target compounds, streamlining the synthesis process.
Accurate Predictions: Utilizing the extensive PISTACHIO, BKMS_METABOLIC, PISTACHIO_RINGBREAKER, REAXYS, REAXYS_BIOCATALYSIS database, our tool offers high-accuracy predictions, reflecting the latest in chemical research and data.
Strategy Settings
Precursor scoring | Relevance Heuristic |
---|---|
Min. plausibility | 0.01 |
Model | Template_relevance |
Template Set | Pistachio/Bkms_metabolic/Pistachio_ringbreaker/Reaxys/Reaxys_biocatalysis |
Top-N result to add to graph | 6 |
Feasible Synthetic Routes
Q1: What is the molecular formula and weight of 2,6-Dimethylphenyl isocyanide?
A1: The molecular formula of this compound is C9H9N, and its molecular weight is 131.17 g/mol.
Q2: What spectroscopic techniques are commonly used to characterize this compound and its metal complexes?
A2: Common techniques include Infrared (IR) spectroscopy, Nuclear Magnetic Resonance (NMR) spectroscopy (1H and 13C), and X-ray crystallography. IR spectroscopy provides information about the C≡N stretching frequency, a key indicator of metal-ligand backbonding. NMR spectroscopy elucidates the structure and dynamics of complexes in solution. X-ray crystallography offers detailed structural information in the solid state.
Q3: What types of reactions is this compound known to undergo with metal complexes?
A3: this compound exhibits a rich reaction chemistry with metal complexes, including:
- Coordination: It readily coordinates to various transition metals in different oxidation states. [, , , ]
- Insertion: It can insert into metal-carbon and metal-nitrogen bonds, leading to the formation of iminoacyl, enediamido, and other functionalized complexes. [, , , , ]
- Coupling: It can undergo C-C and C-N coupling reactions, especially in the presence of alkynes and other unsaturated substrates. []
Q4: What influences the selectivity of isocyanide insertion into metal-carbon versus metal-nitrogen bonds?
A4: Both steric and electronic factors contribute to the selectivity:
- Electronic factors: The relative bond strengths of the metal-carbon and metal-nitrogen bonds play a significant role. For instance, in [Ti(η5-Ind)(NMe2)2Me], insertion occurs exclusively into the Ti−C bond due to its weaker nature compared to the Ti−NR2 bond. []
Q5: How does this compound react with diborane compounds?
A6: It inserts into the B-B bond of specific five-membered cyclic organo-1,2-diboranes, yielding unique spirocyclic products. [] The steric bulk of the isocyanide substituents prevents dimerization or rearrangement of the resulting insertion products.
Q6: Can this compound be used to synthesize heterometallic complexes?
A7: Yes, it has been successfully employed in the synthesis of a heterotetranuclear Mo(VI)2Cu(I)2 complex, demonstrating its potential for constructing complex multimetallic architectures. []
Q7: What role does this compound play in studying metal-metal interactions?
A8: The steric bulk of this compound can influence the packing of metal complexes in the solid state. In a series of Pt(II) and Pd(II) complexes, the presence of this ligand disrupts extended metal-metal interactions, highlighting the interplay between ligand sterics and metallophilic bonding. []
Q8: How does this compound contribute to understanding heterogeneous catalysis?
A9: It serves as a sensitive spectroscopic probe for studying heterogeneous nucleation and deposition processes on metal surfaces. [] Its C≡N stretching frequency is sensitive to the nature of the metal it is bound to and the coordination mode, allowing for atomic-level tracking of surface reactions.
Q9: Can you elaborate on the use of this compound in studying surface-enhanced Raman scattering (SERS)?
A10: The C≡N stretching frequency of this compound is significantly enhanced on metal surfaces, making it an effective SERS probe. This property was exploited to investigate the surface composition of Au/Ag alloy nanoparticles, revealing a surface enrichment of Ag atoms. []
Q10: How is computational chemistry used to study this compound and its metal complexes?
A10: Density functional theory (DFT) calculations are frequently employed to:
- Investigate bonding: Understand the nature of metal-ligand interactions and the electronic structure of complexes. [, , , ]
- Explore reaction mechanisms: Elucidate the pathways involved in isocyanide insertion, coupling, and other reactions. [, , , ]
- Predict properties: Calculate vibrational frequencies, NMR chemical shifts, and other spectroscopic parameters to aid in the characterization of complexes. [, ]
Q11: Have there been studies on modifying the structure of this compound to tune its properties?
A12: Yes, researchers have explored the effects of varying the aryl substituents on the isocyanide ligand. For example, incorporating electron-donating or -withdrawing groups on the phenyl ring can modulate the electron density at the metal center and influence reactivity. [, , ]
Disclaimer and Information on In-Vitro Research Products
Please be aware that all articles and product information presented on BenchChem are intended solely for informational purposes. The products available for purchase on BenchChem are specifically designed for in-vitro studies, which are conducted outside of living organisms. In-vitro studies, derived from the Latin term "in glass," involve experiments performed in controlled laboratory settings using cells or tissues. It is important to note that these products are not categorized as medicines or drugs, and they have not received approval from the FDA for the prevention, treatment, or cure of any medical condition, ailment, or disease. We must emphasize that any form of bodily introduction of these products into humans or animals is strictly prohibited by law. It is essential to adhere to these guidelines to ensure compliance with legal and ethical standards in research and experimentation.