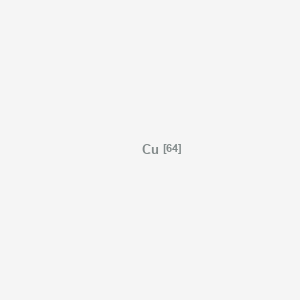
Copper, isotope of mass 64
Overview
Description
Copper-64 is a radioisotope of copper with a half-life of approximately 12.7 hours. It emits both positrons and beta particles, making it useful for both diagnostic and therapeutic applications. Copper-64 is particularly valuable in positron emission tomography (PET) imaging and molecular radiotherapy due to its unique decay properties .
Preparation Methods
Copper-64 can be produced using several methods, primarily involving nuclear reactions. The most common methods include:
Reactor Production: Copper-64 can be produced by irradiating copper-63 with thermal neutrons, resulting in the reaction ({64}Cu).
Cyclotron Production: High specific activity Copper-64 can be produced by irradiating nickel-64 with protons, resulting in the reaction ({64}Cu).
Alternative Methods: Other methods include the irradiation of zinc-64 with high-energy neutrons, resulting in the reaction ({64}Zn(n,p){64}Cu).
Chemical Reactions Analysis
Copper-64 undergoes various chemical reactions, including:
Oxidation: Copper-64 can be oxidized to form copper(II) ions. For example, in the presence of sulfuric acid, copper-64 reacts to form copper(II) sulfate and hydrogen gas[ Cu + 2H_2SO_4 \rightarrow CuSO_4 + SO_2 + 2H_2O ]
Reduction: Copper-64 can be reduced by stronger reducing agents such as zinc, forming copper metal and zinc ions[ Cu^{2+} + Zn \rightarrow Cu + Zn^{2+} ]
Substitution: Copper-64 can undergo substitution reactions with halogens to form copper halides. For example, with chlorine gas, it forms copper(II) chloride[ Cu + Cl_2 \rightarrow CuCl_2 ]
Scientific Research Applications
Copper-64 has a wide range of scientific research applications:
Medical Imaging: Copper-64 is extensively used in PET imaging to diagnose various conditions, including cancer and neuroendocrine tumors.
Radiotherapy: Due to its beta emissions, Copper-64 is used in targeted radiotherapy to treat cancer.
Biological Research: Copper-64 is used to study copper metabolism and its role in various biological processes.
Industrial Applications: Copper-64 is used in industrial radiography and tracer studies to investigate the behavior of materials and processes.
Mechanism of Action
Copper-64 exerts its effects primarily through its radioactive decay. In PET imaging, the positrons emitted by Copper-64 interact with electrons in the body, producing gamma rays that are detected by the PET scanner. This allows for high-resolution imaging of biological processes .
In radiotherapy, the beta particles emitted by Copper-64 cause damage to the DNA of cancer cells, leading to cell death. The specific targeting of Copper-64 to cancer cells is achieved by attaching it to molecules that bind to receptors overexpressed on the surface of these cells .
Comparison with Similar Compounds
Copper-64 is often compared with other radioisotopes used in medical imaging and therapy, such as:
Fluorine-18: Fluorine-18 is another positron-emitting isotope used in PET imaging.
Gallium-68: Gallium-68 is used in PET imaging for similar applications as Copper-64.
Copper-67: Copper-67 is a beta and gamma-emitting isotope used in radiotherapy.
Copper-64’s unique combination of positron and beta emissions, along with its suitable half-life, makes it a versatile and valuable isotope in both diagnostic and therapeutic applications.
Properties
CAS No. |
13981-25-4 |
---|---|
Molecular Formula |
Cu |
Molecular Weight |
63.929764 g/mol |
IUPAC Name |
copper-64 |
InChI |
InChI=1S/Cu/i1+0 |
InChI Key |
RYGMFSIKBFXOCR-IGMARMGPSA-N |
Isomeric SMILES |
[64Cu] |
SMILES |
[Cu] |
Canonical SMILES |
[Cu] |
Synonyms |
64Cu radioisotope Copper-64 Cu-64 radioisotope |
Origin of Product |
United States |
Synthesis routes and methods I
Procedure details
Synthesis routes and methods II
Procedure details
Synthesis routes and methods III
Procedure details
Synthesis routes and methods IV
Procedure details
Disclaimer and Information on In-Vitro Research Products
Please be aware that all articles and product information presented on BenchChem are intended solely for informational purposes. The products available for purchase on BenchChem are specifically designed for in-vitro studies, which are conducted outside of living organisms. In-vitro studies, derived from the Latin term "in glass," involve experiments performed in controlled laboratory settings using cells or tissues. It is important to note that these products are not categorized as medicines or drugs, and they have not received approval from the FDA for the prevention, treatment, or cure of any medical condition, ailment, or disease. We must emphasize that any form of bodily introduction of these products into humans or animals is strictly prohibited by law. It is essential to adhere to these guidelines to ensure compliance with legal and ethical standards in research and experimentation.