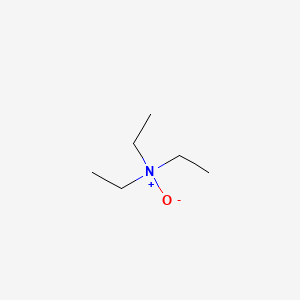
Triethylamine N-oxide
Overview
Description
Triethylamine N-oxide is an organic compound that belongs to the class of amine oxides. It is a colorless solid that is soluble in water and various organic solvents. This compound is primarily used as a reagent in organic synthesis and has applications in various scientific fields.
Mechanism of Action
Target of Action
Triethylamine N-oxide, also known as Ethanamine, N,N-diethyl-, N-oxide or N,N-diethylethanamine oxide, primarily targets proteins in the body . It serves as a protein stabilizer, counteracting the protein-destabilizing effects of pressure . This compound is closely associated with cellular aging and age-related diseases . It can accelerate cell senescence by causing mitochondrial damage, superoxide formation, and promoting the generation of pro-inflammatory factors .
Mode of Action
this compound interacts with its targets, the proteins, by stabilizing them against the destabilizing effects of pressure . This stabilization is particularly crucial for marine organisms living at great depths, where high pressure could otherwise distort proteins and cause harm .
Biochemical Pathways
this compound is primarily derived from the gut, where the gut microbiota converts trimethylamine precursors into trimethylamine. This is then absorbed into the bloodstream through the intestinal mucosa and transformed into this compound by hepatic flavin monooxygenases (FMOs) in the liver . This compound can also be synthesized from trimethylamine by treatment with hydrogen peroxide .
Pharmacokinetics
this compound is efficiently absorbed from the gastrointestinal tract, rapidly distributed, and partly metabolized . It is also well absorbed from the gastrointestinal tract. Within the gastrointestinal tract, this compound is reduced into triethylamine (19%) and dealkylated into diethylamine (10%) . The apparent volumes of distribution during the elimination phase are 192 liters for triethylamine and 103 liters for this compound . More than 90% of the dose is recovered in the urine as triethylamine and this compound .
Result of Action
The primary result of this compound’s action is the stabilization of proteins, particularly under conditions of high pressure . This stabilization is crucial for the survival of marine organisms living at great depths . In addition, this compound has been associated with cellular aging and age-related diseases . It can accelerate cell senescence by causing mitochondrial damage, superoxide formation, and promoting the generation of pro-inflammatory factors .
Action Environment
The action of this compound is influenced by environmental factors. For instance, the concentration of this compound increases with the depth at which marine organisms live, reflecting the increased need for protein stabilization under high-pressure conditions . Furthermore, dietary factors can influence the levels of this compound in the body. Diets rich in animal protein such as eggs, red meat, shellfish, and total fish consumption can increase this compound levels , while plant-based diets such as vegan, vegetarian, and the Mediterranean diet can lower this compound levels .
Preparation Methods
Synthetic Routes and Reaction Conditions: Triethylamine N-oxide can be synthesized through the oxidation of triethylamine. The most common oxidizing agents used for this purpose are hydrogen peroxide and peroxycarboxylic acids. The reaction typically proceeds under mild conditions, with the triethylamine being dissolved in an appropriate solvent and the oxidizing agent being added slowly to control the reaction rate .
Industrial Production Methods: In industrial settings, the production of this compound follows similar principles but on a larger scale. The process involves the continuous addition of triethylamine and the oxidizing agent in a controlled environment to ensure safety and efficiency. The product is then purified through crystallization or distillation to obtain the desired purity.
Chemical Reactions Analysis
Types of Reactions: Triethylamine N-oxide undergoes various chemical reactions, including:
Oxidation: It can be further oxidized to produce different nitrogen oxides.
Reduction: It can be reduced back to triethylamine under specific conditions.
Substitution: It can participate in substitution reactions where the oxygen atom is replaced by other functional groups.
Common Reagents and Conditions:
Oxidation: Hydrogen peroxide or peroxycarboxylic acids are commonly used.
Reduction: Reducing agents like lithium aluminum hydride can be employed.
Substitution: Various nucleophiles can be used depending on the desired product.
Major Products Formed:
Oxidation: Higher nitrogen oxides.
Reduction: Triethylamine.
Substitution: Various substituted amine oxides depending on the nucleophile used.
Scientific Research Applications
Triethylamine N-oxide has a wide range of applications in scientific research:
Chemistry: It is used as a reagent in organic synthesis, particularly in oxidation reactions.
Biology: It is used in studies involving protein folding and stability.
Industry: It is used in the production of various chemicals and materials.
Comparison with Similar Compounds
Trimethylamine N-oxide: Another amine oxide with similar properties but different applications.
Diethylamine N-oxide: A related compound with two ethyl groups instead of three.
Uniqueness: Triethylamine N-oxide is unique due to its specific structure and reactivity. Its three ethyl groups provide distinct steric and electronic properties, making it suitable for specific reactions and applications that other amine oxides may not be able to perform as effectively .
Properties
IUPAC Name |
N,N-diethylethanamine oxide | |
---|---|---|
Source | PubChem | |
URL | https://pubchem.ncbi.nlm.nih.gov | |
Description | Data deposited in or computed by PubChem | |
InChI |
InChI=1S/C6H15NO/c1-4-7(8,5-2)6-3/h4-6H2,1-3H3 | |
Source | PubChem | |
URL | https://pubchem.ncbi.nlm.nih.gov | |
Description | Data deposited in or computed by PubChem | |
InChI Key |
LFMTUFVYMCDPGY-UHFFFAOYSA-N | |
Source | PubChem | |
URL | https://pubchem.ncbi.nlm.nih.gov | |
Description | Data deposited in or computed by PubChem | |
Canonical SMILES |
CC[N+](CC)(CC)[O-] | |
Source | PubChem | |
URL | https://pubchem.ncbi.nlm.nih.gov | |
Description | Data deposited in or computed by PubChem | |
Molecular Formula |
C6H15NO | |
Source | PubChem | |
URL | https://pubchem.ncbi.nlm.nih.gov | |
Description | Data deposited in or computed by PubChem | |
DSSTOX Substance ID |
DTXSID1062592 | |
Record name | Ethanamine, N,N-diethyl-, N-oxide | |
Source | EPA DSSTox | |
URL | https://comptox.epa.gov/dashboard/DTXSID1062592 | |
Description | DSSTox provides a high quality public chemistry resource for supporting improved predictive toxicology. | |
Molecular Weight |
117.19 g/mol | |
Source | PubChem | |
URL | https://pubchem.ncbi.nlm.nih.gov | |
Description | Data deposited in or computed by PubChem | |
CAS No. |
2687-45-8 | |
Record name | Ethanamine, N,N-diethyl-, N-oxide | |
Source | CAS Common Chemistry | |
URL | https://commonchemistry.cas.org/detail?cas_rn=2687-45-8 | |
Description | CAS Common Chemistry is an open community resource for accessing chemical information. Nearly 500,000 chemical substances from CAS REGISTRY cover areas of community interest, including common and frequently regulated chemicals, and those relevant to high school and undergraduate chemistry classes. This chemical information, curated by our expert scientists, is provided in alignment with our mission as a division of the American Chemical Society. | |
Explanation | The data from CAS Common Chemistry is provided under a CC-BY-NC 4.0 license, unless otherwise stated. | |
Record name | Triethylamine-N-oxide | |
Source | ChemIDplus | |
URL | https://pubchem.ncbi.nlm.nih.gov/substance/?source=chemidplus&sourceid=0002687458 | |
Description | ChemIDplus is a free, web search system that provides access to the structure and nomenclature authority files used for the identification of chemical substances cited in National Library of Medicine (NLM) databases, including the TOXNET system. | |
Record name | Ethanamine, N,N-diethyl-, N-oxide | |
Source | EPA Chemicals under the TSCA | |
URL | https://www.epa.gov/chemicals-under-tsca | |
Description | EPA Chemicals under the Toxic Substances Control Act (TSCA) collection contains information on chemicals and their regulations under TSCA, including non-confidential content from the TSCA Chemical Substance Inventory and Chemical Data Reporting. | |
Record name | Ethanamine, N,N-diethyl-, N-oxide | |
Source | EPA DSSTox | |
URL | https://comptox.epa.gov/dashboard/DTXSID1062592 | |
Description | DSSTox provides a high quality public chemistry resource for supporting improved predictive toxicology. | |
Record name | Triethylamine oxide | |
Source | European Chemicals Agency (ECHA) | |
URL | https://echa.europa.eu/information-on-chemicals | |
Description | The European Chemicals Agency (ECHA) is an agency of the European Union which is the driving force among regulatory authorities in implementing the EU's groundbreaking chemicals legislation for the benefit of human health and the environment as well as for innovation and competitiveness. | |
Explanation | Use of the information, documents and data from the ECHA website is subject to the terms and conditions of this Legal Notice, and subject to other binding limitations provided for under applicable law, the information, documents and data made available on the ECHA website may be reproduced, distributed and/or used, totally or in part, for non-commercial purposes provided that ECHA is acknowledged as the source: "Source: European Chemicals Agency, http://echa.europa.eu/". Such acknowledgement must be included in each copy of the material. ECHA permits and encourages organisations and individuals to create links to the ECHA website under the following cumulative conditions: Links can only be made to webpages that provide a link to the Legal Notice page. | |
Synthesis routes and methods
Procedure details
Q1: What is the metabolism of Triethylamine, and how is Triethylamine N-oxide involved?
A1: Triethylamine (TEA) is primarily metabolized to this compound (TEAO) in humans. Studies show that approximately 24% [] of inhaled TEA is converted to TEAO, with a wide interindividual variation (15-36%) []. This metabolic process makes urinary TEA and TEAO levels good indicators of TEA exposure [, ].
Q2: Can you elaborate on the use of this compound and Triethylamine as biomarkers for occupational exposure?
A2: Both Triethylamine (TEA) and its metabolite, this compound (TEAO), are valuable biomarkers for assessing occupational exposure to TEA. Studies involving workers in polyurethane foam production, where TEA is commonly used, have demonstrated a strong correlation between TEA air levels and urinary concentrations of TEA and TEAO []. Specifically, post-shift urine and plasma TEA levels effectively reflect the time-weighted average TEA concentration in the air during the workday [].
Q3: What is the significance of the bond dissociation energy (BDE) of the N-O bond in this compound?
A3: The N-O bond dissociation energy (BDE) in this compound is crucial for understanding its reactivity and stability. Computational studies using methods like CBS-QB3, CBS-APNO, G4, and M06-2X/6-311+G(3df,2p) DFT have revealed that the N-O single-bond BDEs in trialkylamine oxides, including this compound, are higher than previously thought []. This finding challenges the generic N-O BDE of 48 kcal/mol often cited in the literature []. Accurate BDE values are essential for predicting reactivity and designing new synthetic strategies involving these compounds.
Q4: Besides its role as a metabolite, does this compound have any notable applications in chemistry?
A4: Yes, this compound has found application as a mild and selective reagent for the deprotection of tert-butyldimethylsilyl (TBS) ethers from phenols []. This method offers a valuable alternative to traditional deprotection techniques, potentially providing advantages in terms of selectivity and reaction conditions.
Disclaimer and Information on In-Vitro Research Products
Please be aware that all articles and product information presented on BenchChem are intended solely for informational purposes. The products available for purchase on BenchChem are specifically designed for in-vitro studies, which are conducted outside of living organisms. In-vitro studies, derived from the Latin term "in glass," involve experiments performed in controlled laboratory settings using cells or tissues. It is important to note that these products are not categorized as medicines or drugs, and they have not received approval from the FDA for the prevention, treatment, or cure of any medical condition, ailment, or disease. We must emphasize that any form of bodily introduction of these products into humans or animals is strictly prohibited by law. It is essential to adhere to these guidelines to ensure compliance with legal and ethical standards in research and experimentation.