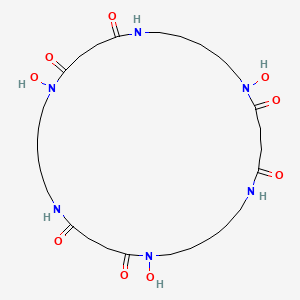
Nocardamine
Overview
Description
Nocardamine is a ferrioxamine siderophore found in Streptomyces and has diverse biological activities . It chelates iron in a chrome azurol S assay (IC 50 = 9.9 µM). Nocardamine inhibits M. smegmatis and M. bovis biofilm formation (MIC = 10 µM for both), an effect that can be reversed by iron . It is cytotoxic to T47D, SK-MEL-5, SK-MEL-28, and RPMI-7951 cancer cells (IC 50 s = 6, 18, 12, and 14 µM, respectively). Nocardamine also induces morphological changes in BM-N4 insect cells .
Synthesis Analysis
The complete genome sequence of marine-derived Streptomyces atratus ZH16 reveals that this strain has the potential to produce nocardamine-type (desferrioxamine) compounds . The activation of an embedded siderophore gene cluster via the application of metabolic engineering methods has been reported .Molecular Structure Analysis
Nocardamine has a molecular formula of C27H48N6O9 . Its average mass is 600.705 Da and its monoisotopic mass is 600.348267 Da .Physical And Chemical Properties Analysis
Nocardamine has a density of 1.2±0.1 g/cm^3 and a molar refractivity of 150.8±0.3 cm^3 . It has 15 H bond acceptors, 6 H bond donors, and 0 freely rotating bonds . Its polar surface area is 209 Å^2 and its molar volume is 516.6±3.0 cm^3 .Scientific Research Applications
Antimalarial Activity
Nocardamine has been identified as an active compound with potent antimalarial activity . It has an IC50 of 1.5 μM, which is 10-fold more potent than Dibutyl Phthalate (DBP), another compound from the same source . The antimalarial activity of Nocardamine is attributed to its iron-chelating ability, which starves the parasites of their iron source, inhibiting their growth .
Oxidative Stress Mitigation
Nocardamine has been shown to mitigate cellular dysfunction induced by oxidative stress in periodontal ligament stem cells (PDLSCs) . It effectively inhibits hydrogen peroxide-induced oxidative stress in PDLSCs, restores alkaline phosphatase activity, mineralized nodule formation, and the expression of osteogenic markers .
Tissue Regeneration
Desferrioxamine E, an iron chelator, has been used in tissue regeneration due to its ability to promote angiogenesis . Blood vessels play a crucial role in sustaining life by facilitating the delivery of immune cells, oxygen, and nutrients, as well as eliminating waste products generated during cellular metabolism . Desferrioxamine E can reduce inflammatory response and promote angiogenesis, which is beneficial for tissue regeneration .
Chronic Wound Healing
Desferrioxamine E has been applied in the healing of chronic wounds . It promotes angiogenesis, which is crucial for wound healing .
Bone Repair
Desferrioxamine E has been used in bone repair . It promotes angiogenesis, which is essential for bone repair .
Respiratory System Diseases
Desferrioxamine E has been used in the treatment of diseases of the respiratory system . It promotes angiogenesis, which is beneficial for the respiratory system .
Iron (III) Detection
Desferrioxamine E has been used in the development of sensors for Fe(III) detection . It forms stable complexes with iron (III) and other divalent and trivalent metal ions .
Drug Delivery
Desferrioxamine E has been used in the development of drug delivery systems . It can be modified or functionalized on solid phases, nanoobjects, biopolymers, electrodes, and optical devices .
Safety and Hazards
Future Directions
Nocardamine has shown potent antimalarial activity with an IC 50 of 1.5 μM . The addition of ≥12.5 µM ferric ions into the Pf culture reduced nocardamine antimalarial activity by 90% under in vitro settings . Hence, the iron-chelating ability of nocardamine was shown to starve the parasites from their iron source, eventually inhibiting their growth . This suggests potential future directions for the use of Nocardamine in antimalarial therapies.
Mechanism of Action
Target of Action
Nocardamine, also known as Desferrioxamine E, is a cyclic siderophore that has been shown to possess anti-cancer and anti-bacterial properties . It has been identified as an active compound with potent antimalarial activity .
Mode of Action
Nocardamine’s mode of action is primarily through its iron-chelating ability . It starves parasites from their iron source, eventually inhibiting their growth . This iron-chelating ability is crucial for its antimalarial activity .
Biochemical Pathways
Nocardamine has been shown to mitigate cellular dysfunction induced by oxidative stress in periodontal ligament stem cells . It effectively inhibits H₂O₂-induced oxidative stress in these cells . It also restores alkaline phosphatase activity, mineralized nodule formation, and the expression of osteogenic markers in H₂O₂-stimulated cells . Mechanistically, nocardamine increases p-ERK level and promotes β-catenin translocation into the nucleus .
Pharmacokinetics
It has been suggested that factors such as bioavailability, pharmacokinetics, and the interaction of nocardamine with the host’s immune system and other physiological processes can be assessed in animal models . These are critical considerations for the development of nocardamine as a clinical therapeutic .
Result of Action
Nocardamine protects periodontal ligament stem cells against H₂O₂-induced oxidative stress and effectively restores impaired osteogenic differentiation in these cells by modulating the ERK/Wnt signaling pathway . It exhibits no significant cytotoxicity at concentrations below 20 µM .
Action Environment
The action of nocardamine can be influenced by environmental factors. For instance, the addition of ≥12.5 µM ferric ions into the Plasmodium falciparum culture reduced nocardamine antimalarial activity by 90% under in vitro settings . This suggests that the presence of ferric ions in the environment can significantly impact the efficacy of nocardamine.
properties
IUPAC Name |
1,12,23-trihydroxy-1,6,12,17,23,28-hexazacyclotritriacontane-2,5,13,16,24,27-hexone | |
---|---|---|
Source | PubChem | |
URL | https://pubchem.ncbi.nlm.nih.gov | |
Description | Data deposited in or computed by PubChem | |
InChI |
InChI=1S/C27H48N6O9/c34-22-10-14-26(38)32(41)20-8-3-6-18-30-24(36)12-15-27(39)33(42)21-9-2-5-17-29-23(35)11-13-25(37)31(40)19-7-1-4-16-28-22/h40-42H,1-21H2,(H,28,34)(H,29,35)(H,30,36) | |
Source | PubChem | |
URL | https://pubchem.ncbi.nlm.nih.gov | |
Description | Data deposited in or computed by PubChem | |
InChI Key |
NHKCCADZVLTPPO-UHFFFAOYSA-N | |
Source | PubChem | |
URL | https://pubchem.ncbi.nlm.nih.gov | |
Description | Data deposited in or computed by PubChem | |
Canonical SMILES |
C1CCNC(=O)CCC(=O)N(CCCCCNC(=O)CCC(=O)N(CCCCCNC(=O)CCC(=O)N(CC1)O)O)O | |
Source | PubChem | |
URL | https://pubchem.ncbi.nlm.nih.gov | |
Description | Data deposited in or computed by PubChem | |
Molecular Formula |
C27H48N6O9 | |
Source | PubChem | |
URL | https://pubchem.ncbi.nlm.nih.gov | |
Description | Data deposited in or computed by PubChem | |
DSSTOX Substance ID |
DTXSID00181161 | |
Record name | Nocardamine | |
Source | EPA DSSTox | |
URL | https://comptox.epa.gov/dashboard/DTXSID00181161 | |
Description | DSSTox provides a high quality public chemistry resource for supporting improved predictive toxicology. | |
Molecular Weight |
600.7 g/mol | |
Source | PubChem | |
URL | https://pubchem.ncbi.nlm.nih.gov | |
Description | Data deposited in or computed by PubChem | |
Product Name |
Nocardamine | |
CAS RN |
26605-16-3 | |
Record name | Desferrioxamine E | |
Source | CAS Common Chemistry | |
URL | https://commonchemistry.cas.org/detail?cas_rn=26605-16-3 | |
Description | CAS Common Chemistry is an open community resource for accessing chemical information. Nearly 500,000 chemical substances from CAS REGISTRY cover areas of community interest, including common and frequently regulated chemicals, and those relevant to high school and undergraduate chemistry classes. This chemical information, curated by our expert scientists, is provided in alignment with our mission as a division of the American Chemical Society. | |
Explanation | The data from CAS Common Chemistry is provided under a CC-BY-NC 4.0 license, unless otherwise stated. | |
Record name | Nocardamin | |
Source | ChemIDplus | |
URL | https://pubchem.ncbi.nlm.nih.gov/substance/?source=chemidplus&sourceid=0026605163 | |
Description | ChemIDplus is a free, web search system that provides access to the structure and nomenclature authority files used for the identification of chemical substances cited in National Library of Medicine (NLM) databases, including the TOXNET system. | |
Record name | Nocardamine | |
Source | EPA DSSTox | |
URL | https://comptox.epa.gov/dashboard/DTXSID00181161 | |
Description | DSSTox provides a high quality public chemistry resource for supporting improved predictive toxicology. | |
Record name | NOCARDAMIN | |
Source | FDA Global Substance Registration System (GSRS) | |
URL | https://gsrs.ncats.nih.gov/ginas/app/beta/substances/Q645668975 | |
Description | The FDA Global Substance Registration System (GSRS) enables the efficient and accurate exchange of information on what substances are in regulated products. Instead of relying on names, which vary across regulatory domains, countries, and regions, the GSRS knowledge base makes it possible for substances to be defined by standardized, scientific descriptions. | |
Explanation | Unless otherwise noted, the contents of the FDA website (www.fda.gov), both text and graphics, are not copyrighted. They are in the public domain and may be republished, reprinted and otherwise used freely by anyone without the need to obtain permission from FDA. Credit to the U.S. Food and Drug Administration as the source is appreciated but not required. | |
Retrosynthesis Analysis
AI-Powered Synthesis Planning: Our tool employs the Template_relevance Pistachio, Template_relevance Bkms_metabolic, Template_relevance Pistachio_ringbreaker, Template_relevance Reaxys, Template_relevance Reaxys_biocatalysis model, leveraging a vast database of chemical reactions to predict feasible synthetic routes.
One-Step Synthesis Focus: Specifically designed for one-step synthesis, it provides concise and direct routes for your target compounds, streamlining the synthesis process.
Accurate Predictions: Utilizing the extensive PISTACHIO, BKMS_METABOLIC, PISTACHIO_RINGBREAKER, REAXYS, REAXYS_BIOCATALYSIS database, our tool offers high-accuracy predictions, reflecting the latest in chemical research and data.
Strategy Settings
Precursor scoring | Relevance Heuristic |
---|---|
Min. plausibility | 0.01 |
Model | Template_relevance |
Template Set | Pistachio/Bkms_metabolic/Pistachio_ringbreaker/Reaxys/Reaxys_biocatalysis |
Top-N result to add to graph | 6 |
Feasible Synthetic Routes
Disclaimer and Information on In-Vitro Research Products
Please be aware that all articles and product information presented on BenchChem are intended solely for informational purposes. The products available for purchase on BenchChem are specifically designed for in-vitro studies, which are conducted outside of living organisms. In-vitro studies, derived from the Latin term "in glass," involve experiments performed in controlled laboratory settings using cells or tissues. It is important to note that these products are not categorized as medicines or drugs, and they have not received approval from the FDA for the prevention, treatment, or cure of any medical condition, ailment, or disease. We must emphasize that any form of bodily introduction of these products into humans or animals is strictly prohibited by law. It is essential to adhere to these guidelines to ensure compliance with legal and ethical standards in research and experimentation.