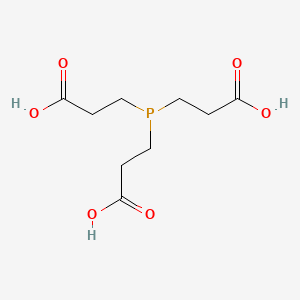
Tris(2-carboxyethyl)phosphine
Overview
Description
Mechanism of Action
Target of Action
The primary target of TCEP is the disulfide bonds within and between proteins . Disulfide bonds play a crucial role in the stability and function of proteins. By targeting these bonds, TCEP can influence protein structure and function .
Mode of Action
TCEP acts as a reducing agent . It interacts with its targets by breaking the disulfide bonds within and between proteins . This interaction results in the reduction of disulfide bonds to two free thiols or cysteines .
Biochemical Pathways
TCEP affects the biochemical pathways involving proteins with disulfide bonds. By reducing these bonds, TCEP can alter the conformation and function of these proteins . This can have downstream effects on various biological processes, including protein folding, enzyme activity, and signal transduction .
Pharmacokinetics
It is known that tcep is soluble in water , which suggests it could be readily absorbed and distributed in the body. Its impact on bioavailability would depend on factors such as dosage, route of administration, and individual patient characteristics.
Result of Action
The result of TCEP’s action is the cleavage of disulfide bonds, leading to changes in protein structure and function . This can have various molecular and cellular effects, depending on the specific proteins and pathways involved .
Action Environment
TCEP exhibits reducing activity at a wide pH range from 1.5 to 9.0 . It is also more resistant to oxidation in air compared to other reducing agents . These properties suggest that TCEP’s action, efficacy, and stability can be influenced by environmental factors such as pH and oxygen levels .
Biochemical Analysis
Biochemical Properties
Tris(2-carboxyethyl)phosphine plays a crucial role in biochemical reactions by reducing disulfide bonds in proteins and peptides. It interacts with enzymes, proteins, and other biomolecules that contain disulfide bonds, such as cysteine residues . The reduction process involves the cleavage of the disulfide bond, resulting in the formation of two free thiol groups . This property makes this compound particularly useful in the preparation of protein samples for various analytical techniques, including mass spectrometry and chromatography .
Cellular Effects
This compound has significant effects on various types of cells and cellular processes. It has been shown to lower reactive oxygen species levels, increase glutathione levels, and enhance mitochondrial content . These effects contribute to its anti-cancer properties, as it can influence cell signaling pathways, gene expression, and cellular metabolism . This compound’s ability to maintain a reducing environment within cells is critical for preserving protein function and preventing oxidative damage .
Molecular Mechanism
The molecular mechanism of this compound involves the reduction of disulfide bonds through nucleophilic substitution by the phosphorus atom . The phosphorus atom attacks one sulfur atom along the disulfide bond, forming a thioalkoxyphosphonium cation and a sulfhydryl anion . Rapid hydrolysis then releases the second sulfhydryl molecule and the phosphine oxide . This mechanism ensures the complete and irreversible reduction of disulfide bonds, making this compound a highly effective reducing agent .
Temporal Effects in Laboratory Settings
In laboratory settings, the effects of this compound can change over time. It is stable in aqueous solutions and does not undergo rapid oxidation, which is a common issue with other reducing agents . Some side reactions have been reported, such as the conversion of cysteine residues into alanine in the presence of heat . Long-term studies have shown that this compound can maintain its reducing activity over extended periods, making it suitable for long-term storage and use in various biochemical applications .
Dosage Effects in Animal Models
The effects of this compound vary with different dosages in animal models. In a study involving an optic nerve crush model in rats, this compound demonstrated neuroprotective effects at a concentration of 100 µM . Higher doses may lead to toxic or adverse effects, highlighting the importance of optimizing dosage for specific applications . The compound’s ability to protect retinal ganglion cells suggests its potential for therapeutic use in neurodegenerative diseases .
Metabolic Pathways
This compound is involved in metabolic pathways that include the reduction of disulfide bonds in proteins and peptides . It interacts with enzymes and cofactors that facilitate these reactions, ensuring the proper folding and function of proteins . The compound’s role in maintaining a reducing environment is essential for various cellular processes, including metabolism and signal transduction .
Transport and Distribution
Within cells and tissues, this compound is transported and distributed through interactions with transporters and binding proteins . Its hydrophilic nature allows it to dissolve easily in aqueous environments, facilitating its distribution throughout the cell . The compound’s ability to maintain its reducing activity in different cellular compartments is crucial for its effectiveness in biochemical applications .
Subcellular Localization
This compound is localized in various subcellular compartments where it exerts its reducing effects . It can target specific compartments or organelles through post-translational modifications and targeting signals . This localization is essential for its role in maintaining the redox balance within cells and ensuring the proper function of proteins and enzymes .
Preparation Methods
Tris(2-carboxyethyl)phosphine can be synthesized through the acid hydrolysis of tris(cyanoethyl)phosphine in refluxing aqueous hydrochloric acid . The reaction involves the following steps:
Alkylation Reaction: Tetrakisphosphonium chloride undergoes an alkylation reaction with a suitable compound in the presence of an organic solvent at a temperature range of 30-60°C.
Hydrolysis Reaction: The resulting compound from the alkylation reaction is then subjected to hydrolysis to yield this compound hydrochloride.
Chemical Reactions Analysis
Tris(2-carboxyethyl)phosphine primarily undergoes reduction reactions. It is highly effective in cleaving disulfide bonds in proteins and peptides . The common reagents and conditions used in these reactions include:
Reduction of Disulfides: This compound reduces disulfide bonds to free thiols under mild conditions.
Reduction of Sulfoxides, Sulfonyl Chlorides, N-oxides, and Azides: It can also reduce these functional groups, making it a versatile reducing agent.
Reaction with Maleimides: This compound is useful in labeling cysteine residues with maleimides, preventing the formation of disulfide bonds.
Scientific Research Applications
Tris(2-carboxyethyl)phosphine has a wide range of applications in scientific research:
Biochemistry and Molecular Biology: It is used to reduce disulfide bonds in proteins and peptides, facilitating their analysis and characterization.
Protein Labeling and Conjugation: The compound is employed in labeling cysteine residues with maleimides, which is crucial for studying protein structure and function.
RNA Isolation: This compound is used in the tissue homogenization process for RNA isolation, ensuring the integrity of RNA samples.
Mass Spectrometry: It is preferred in mass spectrometry applications due to its stability and lack of interference with absorbance measurements.
Comparison with Similar Compounds
Tris(2-carboxyethyl)phosphine is often compared with other reducing agents such as dithiothreitol and β-mercaptoethanol. Here are some key differences:
Odor: Unlike dithiothreitol and β-mercaptoethanol, this compound is odorless, making it more user-friendly.
Stability: This compound is more stable in aqueous solutions and less prone to oxidation compared to dithiothreitol.
Reactivity: It is a more powerful and irreversible reducing agent, ensuring complete reduction of disulfide bonds.
Hydrophilicity: This compound is more hydrophilic, which enhances its solubility and effectiveness in aqueous environments.
Similar compounds include:
- Dithiothreitol (DTT)
- β-Mercaptoethanol
- 2-Mercaptoethylamine
These compounds share similar reducing properties but differ in terms of odor, stability, and reactivity.
Properties
IUPAC Name |
3-[bis(2-carboxyethyl)phosphanyl]propanoic acid | |
---|---|---|
Source | PubChem | |
URL | https://pubchem.ncbi.nlm.nih.gov | |
Description | Data deposited in or computed by PubChem | |
InChI |
InChI=1S/C9H15O6P/c10-7(11)1-4-16(5-2-8(12)13)6-3-9(14)15/h1-6H2,(H,10,11)(H,12,13)(H,14,15) | |
Source | PubChem | |
URL | https://pubchem.ncbi.nlm.nih.gov | |
Description | Data deposited in or computed by PubChem | |
InChI Key |
PZBFGYYEXUXCOF-UHFFFAOYSA-N | |
Source | PubChem | |
URL | https://pubchem.ncbi.nlm.nih.gov | |
Description | Data deposited in or computed by PubChem | |
Canonical SMILES |
C(CP(CCC(=O)O)CCC(=O)O)C(=O)O | |
Source | PubChem | |
URL | https://pubchem.ncbi.nlm.nih.gov | |
Description | Data deposited in or computed by PubChem | |
Molecular Formula |
C9H15O6P | |
Source | PubChem | |
URL | https://pubchem.ncbi.nlm.nih.gov | |
Description | Data deposited in or computed by PubChem | |
DSSTOX Substance ID |
DTXSID90208290 | |
Record name | Tris(2-carboxyethyl)phosphine | |
Source | EPA DSSTox | |
URL | https://comptox.epa.gov/dashboard/DTXSID90208290 | |
Description | DSSTox provides a high quality public chemistry resource for supporting improved predictive toxicology. | |
Molecular Weight |
250.19 g/mol | |
Source | PubChem | |
URL | https://pubchem.ncbi.nlm.nih.gov | |
Description | Data deposited in or computed by PubChem | |
CAS No. |
5961-85-3 | |
Record name | Tris(2-carboxyethyl)phosphine | |
Source | CAS Common Chemistry | |
URL | https://commonchemistry.cas.org/detail?cas_rn=5961-85-3 | |
Description | CAS Common Chemistry is an open community resource for accessing chemical information. Nearly 500,000 chemical substances from CAS REGISTRY cover areas of community interest, including common and frequently regulated chemicals, and those relevant to high school and undergraduate chemistry classes. This chemical information, curated by our expert scientists, is provided in alignment with our mission as a division of the American Chemical Society. | |
Explanation | The data from CAS Common Chemistry is provided under a CC-BY-NC 4.0 license, unless otherwise stated. | |
Record name | Tris(2-carboxyethyl)phosphine | |
Source | ChemIDplus | |
URL | https://pubchem.ncbi.nlm.nih.gov/substance/?source=chemidplus&sourceid=0005961853 | |
Description | ChemIDplus is a free, web search system that provides access to the structure and nomenclature authority files used for the identification of chemical substances cited in National Library of Medicine (NLM) databases, including the TOXNET system. | |
Record name | Tris(2-carboxyethyl)phosphine | |
Source | EPA DSSTox | |
URL | https://comptox.epa.gov/dashboard/DTXSID90208290 | |
Description | DSSTox provides a high quality public chemistry resource for supporting improved predictive toxicology. | |
Record name | TRIS(2-CARBOXYETHYL)PHOSPHINE | |
Source | FDA Global Substance Registration System (GSRS) | |
URL | https://gsrs.ncats.nih.gov/ginas/app/beta/substances/22OAC2MO2S | |
Description | The FDA Global Substance Registration System (GSRS) enables the efficient and accurate exchange of information on what substances are in regulated products. Instead of relying on names, which vary across regulatory domains, countries, and regions, the GSRS knowledge base makes it possible for substances to be defined by standardized, scientific descriptions. | |
Explanation | Unless otherwise noted, the contents of the FDA website (www.fda.gov), both text and graphics, are not copyrighted. They are in the public domain and may be republished, reprinted and otherwise used freely by anyone without the need to obtain permission from FDA. Credit to the U.S. Food and Drug Administration as the source is appreciated but not required. | |
Synthesis routes and methods
Procedure details
Retrosynthesis Analysis
AI-Powered Synthesis Planning: Our tool employs the Template_relevance Pistachio, Template_relevance Bkms_metabolic, Template_relevance Pistachio_ringbreaker, Template_relevance Reaxys, Template_relevance Reaxys_biocatalysis model, leveraging a vast database of chemical reactions to predict feasible synthetic routes.
One-Step Synthesis Focus: Specifically designed for one-step synthesis, it provides concise and direct routes for your target compounds, streamlining the synthesis process.
Accurate Predictions: Utilizing the extensive PISTACHIO, BKMS_METABOLIC, PISTACHIO_RINGBREAKER, REAXYS, REAXYS_BIOCATALYSIS database, our tool offers high-accuracy predictions, reflecting the latest in chemical research and data.
Strategy Settings
Precursor scoring | Relevance Heuristic |
---|---|
Min. plausibility | 0.01 |
Model | Template_relevance |
Template Set | Pistachio/Bkms_metabolic/Pistachio_ringbreaker/Reaxys/Reaxys_biocatalysis |
Top-N result to add to graph | 6 |
Feasible Synthetic Routes
Disclaimer and Information on In-Vitro Research Products
Please be aware that all articles and product information presented on BenchChem are intended solely for informational purposes. The products available for purchase on BenchChem are specifically designed for in-vitro studies, which are conducted outside of living organisms. In-vitro studies, derived from the Latin term "in glass," involve experiments performed in controlled laboratory settings using cells or tissues. It is important to note that these products are not categorized as medicines or drugs, and they have not received approval from the FDA for the prevention, treatment, or cure of any medical condition, ailment, or disease. We must emphasize that any form of bodily introduction of these products into humans or animals is strictly prohibited by law. It is essential to adhere to these guidelines to ensure compliance with legal and ethical standards in research and experimentation.