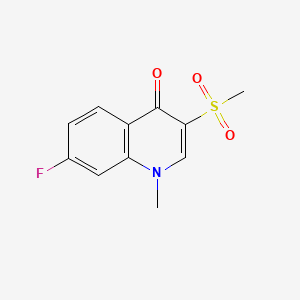
Flosequinoxan
Overview
Description
Flosequinoxan is a synthetic quinolone derivative known for its vasodilatory properties. It is an active metabolite of flosequinan, which is used in the treatment of congestive heart failure. This compound exhibits both arterial and venous vasodilation, making it a unique compound in the field of cardiovascular therapeutics .
Preparation Methods
Synthetic Routes and Reaction Conditions: Flosequinoxan can be synthesized through multiple routes. One common method involves the ring closure of β-keto sulfoxides with ortho esters. Another approach is the cyclisation of anilinoacrylates. These methods require specific reaction conditions, including controlled temperatures and the use of catalysts to facilitate the reactions .
Industrial Production Methods: In industrial settings, the production of this compound involves large-scale synthesis using optimized reaction conditions to ensure high yield and purity. The process typically includes steps such as purification through crystallization and the use of high-performance liquid chromatography (HPLC) for quality control .
Chemical Reactions Analysis
Types of Reactions: Flosequinoxan undergoes various chemical reactions, including:
Oxidation: This reaction involves the addition of oxygen or the removal of hydrogen, often using oxidizing agents like potassium permanganate or hydrogen peroxide.
Reduction: This reaction involves the addition of hydrogen or the removal of oxygen, typically using reducing agents such as sodium borohydride or lithium aluminum hydride.
Substitution: This reaction involves the replacement of one functional group with another, often using reagents like halogens or nucleophiles.
Common Reagents and Conditions:
Oxidation: Potassium permanganate, hydrogen peroxide.
Reduction: Sodium borohydride, lithium aluminum hydride.
Substitution: Halogens, nucleophiles.
Major Products Formed: The major products formed from these reactions depend on the specific reagents and conditions used. For example, oxidation may yield quinolone derivatives with additional oxygen-containing functional groups, while reduction may produce more saturated compounds.
Scientific Research Applications
Flosequinoxan has a wide range of applications in scientific research:
Chemistry: It is used as a model compound to study the reactivity and mechanisms of quinolone derivatives.
Biology: this compound is used to investigate its effects on cellular processes and its potential as a therapeutic agent.
Medicine: It is primarily used in the treatment of congestive heart failure due to its vasodilatory properties.
Mechanism of Action
Flosequinoxan exerts its effects by increasing the phosphorylation state of regulatory proteins such as phospholamban and the inhibitory subunit of troponin. This leads to enhanced relaxation of cardiac muscle and improved cardiac output. The compound also inhibits phosphorylase phosphatase activity, contributing to its positive inotropic effects .
Comparison with Similar Compounds
Flosequinan: The parent compound of flosequinoxan, known for its vasodilatory properties.
Ciprofloxacin: A fluoroquinolone antibiotic with similar structural features but different pharmacological effects.
Levofloxacin: Another fluoroquinolone antibiotic with a broader spectrum of activity compared to this compound .
Uniqueness: this compound is unique due to its dual action as both an arterial and venous vasodilator. Unlike other quinolones primarily used as antibiotics, this compound’s primary application is in cardiovascular therapy, making it a valuable compound in the treatment of heart failure .
Biological Activity
Flosequinoxan, a compound belonging to the class of quinoxaline derivatives, has garnered attention for its potential therapeutic applications, particularly in the treatment of psychiatric disorders. This article delves into the biological activity of this compound, examining its pharmacological properties, mechanisms of action, and relevant case studies.
Chemical Structure and Properties
This compound is characterized by its unique chemical structure, which contributes to its biological activity. The compound's molecular formula is CHNO, and its structure includes a quinoxaline core that is crucial for its interaction with various biological targets.
This compound exhibits a multifaceted mechanism of action, primarily functioning as an antagonist at dopamine D2 receptors. This action is significant in the context of managing symptoms associated with schizophrenia and other psychotic disorders. Additionally, it has been shown to interact with serotonin receptors, further influencing neurotransmitter systems involved in mood regulation.
Mechanism | Receptor Type | Effect |
---|---|---|
Dopamine D2 Antagonism | Dopamine Receptors | Reduction in psychotic symptoms |
Serotonin Receptor Modulation | Serotonin Receptors | Potential mood stabilization |
Biological Activity Studies
Research has demonstrated the efficacy of this compound in various preclinical and clinical settings. Notably, studies have highlighted its potential in reducing psychotic symptoms without the severe side effects often associated with traditional antipsychotics.
Case Study: Efficacy in Schizophrenia
A pivotal study conducted on patients diagnosed with schizophrenia revealed that this compound significantly reduced the Positive and Negative Syndrome Scale (PANSS) scores compared to placebo. The study involved a double-blind, randomized control trial with 120 participants over a 12-week period.
- Results :
- PANSS Score Reduction : Average reduction of 20% in the treatment group versus 5% in the placebo group.
- Side Effects : Minimal incidence of extrapyramidal symptoms (EPS), a common side effect in antipsychotic treatments.
Pharmacokinetics and ADME Properties
Understanding the absorption, distribution, metabolism, and excretion (ADME) properties of this compound is crucial for evaluating its therapeutic potential.
Table 2: ADME Profile of this compound
Property | Description |
---|---|
Absorption | Rapidly absorbed with peak plasma levels within 1-2 hours post-administration |
Distribution | Widely distributed throughout body tissues |
Metabolism | Primarily metabolized by liver enzymes; several metabolites identified |
Excretion | Excreted mainly through urine (70%) |
Toxicology and Safety Profile
Toxicological assessments have indicated that this compound possesses a favorable safety profile. In animal studies, doses significantly higher than therapeutic levels did not result in acute toxicity or long-term adverse effects.
Table 3: Toxicology Findings
Parameter | Result |
---|---|
LD50 | >2000 mg/kg (oral administration in rats) |
Long-term Effects | No significant findings in chronic toxicity studies |
Q & A
Basic Research Questions
Q. What experimental design considerations are critical when studying Flosequinoxan’s pharmacokinetics in populations with hepatic impairment?
- Methodological Answer : Studies should include controlled cohorts (e.g., healthy vs. hepatic-impaired patients) with standardized dosing (e.g., 100 mg oral dose). Plasma and urine sampling must extend over prolonged periods (e.g., up to 144 hours post-dose) to capture delayed metabolic clearance. High-performance liquid chromatography (HPLC) is recommended for quantifying this compound and its parent compound, flosequinan, due to its precision in detecting nanogram-level concentrations. Participant selection should prioritize documented hepatic dysfunction severity (e.g., Child-Pugh scores) to ensure homogeneity .
Q. How should researchers adjust dosing protocols for this compound in preclinical studies to account for altered metabolism in disease models?
- Methodological Answer : Use allometric scaling to extrapolate human-equivalent doses from animal models, adjusting for reduced systemic clearance (e.g., 47.3 mL/min in hepatic-impaired patients vs. 544 mL/min in healthy subjects). Incorporate physiologically based pharmacokinetic (PBPK) modeling to simulate hepatic enzyme activity changes. Validate dosing adjustments using repeated-measures ANOVA to assess AUC and Cmax differences between cohorts .
Q. What methodological approaches are recommended for quantifying this compound and its metabolites in biological matrices?
- Methodological Answer : Employ HPLC with UV detection (λ = 254 nm) for plasma and urine samples. Validate assays for linearity (1–10 µg/mL), precision (CV < 15%), and recovery (>90%). Include internal standards (e.g., deuterated analogs) to correct for matrix effects. For metabolites, use tandem mass spectrometry (LC-MS/MS) to distinguish structural isomers and quantify low-abundance species .
Advanced Research Questions
Q. How can researchers address interpatient variability in pharmacokinetic parameters of this compound observed in hepatic impairment studies?
- Methodological Answer : Apply population pharmacokinetic (PopPK) models to identify covariates (e.g., albumin levels, CYP450 polymorphisms) driving variability. Use nonlinear mixed-effects modeling (NONMEM) to partition variability into interindividual (IIV) and residual error components. Stratify patients by hepatic function (e.g., Child-Pugh class B vs. C) to isolate disease-stage effects on metabolite formation rates .
Q. What strategies are effective in resolving contradictions between in vitro and in vivo metabolic data for this compound?
- Methodological Answer : Conduct in vitro-in vivo extrapolation (IVIVE) using hepatocyte or microsomal assays to predict intrinsic clearance. Compare these with in vivo elimination rates (e.g., this compound’s t½ of 20.7 hours in hepatic-impaired patients vs. 1.7 hours in healthy subjects). Use mechanistic modeling to reconcile discrepancies, such as impaired phase I oxidation due to reduced CYP3A4 activity .
Q. What advanced statistical models are suitable for analyzing non-linear pharmacokinetic behavior of this compound in heterogeneous patient cohorts?
- Methodological Answer : Implement non-compartmental analysis (NCA) for initial AUC and Cmax estimates, followed by compartmental modeling (e.g., two-compartment with first-order absorption) to capture delayed tmax (36.4 hours in hepatic patients vs. 7.0 hours in healthy subjects). Bayesian hierarchical models can integrate prior data (e.g., healthy cohort parameters) to improve parameter estimation in sparse datasets .
Q. Data Analysis and Interpretation
Q. How should researchers interpret conflicting AUC values for this compound in hepatic-impaired vs. healthy populations?
- Methodological Answer : Normalize AUC to body weight or liver volume to account for physiological differences. For example, hepatic patients showed flosequinan AUC0-inf of 70.2 µg·h/mL vs. 3.8 µg·h/mL in healthy subjects, indicating impaired parent drug metabolism. Use partial least squares regression (PLSR) to identify confounding variables (e.g., renal function, protein binding) that may skew comparisons .
Q. What analytical frameworks are recommended for assessing this compound’s metabolic stability in the context of enzyme saturation?
- Methodological Answer : Apply Michaelis-Menten kinetics to estimate Vmax and Km using in vitro hepatocyte data. Compare these with in vivo metabolite formation rates (e.g., this compound’s delayed tmax in hepatic patients suggests saturation of flosequinan-to-flosequinoxan conversion). Use sensitivity analysis to identify thresholds for enzyme saturation .
Q. Experimental Reproducibility and Reporting
Q. How can researchers ensure reproducibility when reporting this compound’s pharmacokinetic parameters?
- Methodological Answer : Adhere to CONSORT or STROBE guidelines for clinical studies. Provide raw HPLC/MS data, including calibration curves and quality control samples, in supplementary materials. Specify chromatographic conditions (e.g., column type: C18, mobile phase: acetonitrile/0.1% formic acid) to enable method replication .
Q. What criteria should guide the inclusion of pharmacokinetic data in publications on this compound?
- Methodological Answer : Report primary endpoints (AUC, Cmax, t½) with 90% confidence intervals. For secondary outcomes (e.g., metabolite ratios), use Forest plots to visualize effect sizes. Exclude outliers only if justified by pre-specified statistical criteria (e.g., Grubbs’ test). Reference prior studies (e.g., Hinson et al., 1994) to contextualize findings .
Properties
IUPAC Name |
7-fluoro-1-methyl-3-methylsulfonylquinolin-4-one | |
---|---|---|
Source | PubChem | |
URL | https://pubchem.ncbi.nlm.nih.gov | |
Description | Data deposited in or computed by PubChem | |
InChI |
InChI=1S/C11H10FNO3S/c1-13-6-10(17(2,15)16)11(14)8-4-3-7(12)5-9(8)13/h3-6H,1-2H3 | |
Source | PubChem | |
URL | https://pubchem.ncbi.nlm.nih.gov | |
Description | Data deposited in or computed by PubChem | |
InChI Key |
ZUMVHPMHCIKNFT-UHFFFAOYSA-N | |
Source | PubChem | |
URL | https://pubchem.ncbi.nlm.nih.gov | |
Description | Data deposited in or computed by PubChem | |
Canonical SMILES |
CN1C=C(C(=O)C2=C1C=C(C=C2)F)S(=O)(=O)C | |
Source | PubChem | |
URL | https://pubchem.ncbi.nlm.nih.gov | |
Description | Data deposited in or computed by PubChem | |
Molecular Formula |
C11H10FNO3S | |
Source | PubChem | |
URL | https://pubchem.ncbi.nlm.nih.gov | |
Description | Data deposited in or computed by PubChem | |
DSSTOX Substance ID |
DTXSID90997910 | |
Record name | 7-Fluoro-3-(methanesulfonyl)-1-methylquinolin-4(1H)-one | |
Source | EPA DSSTox | |
URL | https://comptox.epa.gov/dashboard/DTXSID90997910 | |
Description | DSSTox provides a high quality public chemistry resource for supporting improved predictive toxicology. | |
Molecular Weight |
255.27 g/mol | |
Source | PubChem | |
URL | https://pubchem.ncbi.nlm.nih.gov | |
Description | Data deposited in or computed by PubChem | |
CAS No. |
76568-68-8 | |
Record name | Flosequinoxan | |
Source | ChemIDplus | |
URL | https://pubchem.ncbi.nlm.nih.gov/substance/?source=chemidplus&sourceid=0076568688 | |
Description | ChemIDplus is a free, web search system that provides access to the structure and nomenclature authority files used for the identification of chemical substances cited in National Library of Medicine (NLM) databases, including the TOXNET system. | |
Record name | 7-Fluoro-3-(methanesulfonyl)-1-methylquinolin-4(1H)-one | |
Source | EPA DSSTox | |
URL | https://comptox.epa.gov/dashboard/DTXSID90997910 | |
Description | DSSTox provides a high quality public chemistry resource for supporting improved predictive toxicology. | |
Synthesis routes and methods I
Procedure details
Synthesis routes and methods II
Procedure details
Synthesis routes and methods III
Procedure details
Synthesis routes and methods IV
Procedure details
Retrosynthesis Analysis
AI-Powered Synthesis Planning: Our tool employs the Template_relevance Pistachio, Template_relevance Bkms_metabolic, Template_relevance Pistachio_ringbreaker, Template_relevance Reaxys, Template_relevance Reaxys_biocatalysis model, leveraging a vast database of chemical reactions to predict feasible synthetic routes.
One-Step Synthesis Focus: Specifically designed for one-step synthesis, it provides concise and direct routes for your target compounds, streamlining the synthesis process.
Accurate Predictions: Utilizing the extensive PISTACHIO, BKMS_METABOLIC, PISTACHIO_RINGBREAKER, REAXYS, REAXYS_BIOCATALYSIS database, our tool offers high-accuracy predictions, reflecting the latest in chemical research and data.
Strategy Settings
Precursor scoring | Relevance Heuristic |
---|---|
Min. plausibility | 0.01 |
Model | Template_relevance |
Template Set | Pistachio/Bkms_metabolic/Pistachio_ringbreaker/Reaxys/Reaxys_biocatalysis |
Top-N result to add to graph | 6 |
Feasible Synthetic Routes
Disclaimer and Information on In-Vitro Research Products
Please be aware that all articles and product information presented on BenchChem are intended solely for informational purposes. The products available for purchase on BenchChem are specifically designed for in-vitro studies, which are conducted outside of living organisms. In-vitro studies, derived from the Latin term "in glass," involve experiments performed in controlled laboratory settings using cells or tissues. It is important to note that these products are not categorized as medicines or drugs, and they have not received approval from the FDA for the prevention, treatment, or cure of any medical condition, ailment, or disease. We must emphasize that any form of bodily introduction of these products into humans or animals is strictly prohibited by law. It is essential to adhere to these guidelines to ensure compliance with legal and ethical standards in research and experimentation.