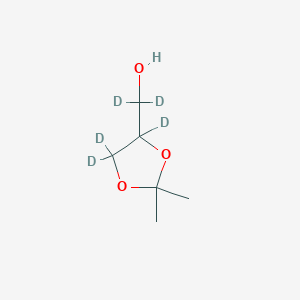
(R,S)-2,2-Dimethyl-1,3-dioxolane-4-methanol-d5
Overview
Description
Synthesis Analysis
The synthesis of compounds similar to (R,S)-2,2-Dimethyl-1,3-dioxolane-4-methanol-d5 has been explored through various methods. One study describes the synthesis and crystal structure of a related compound, indicating detailed crystallization processes and configurations of the molecule (Li, Wang, & Chen, 2001). Another research effort reports the synthesis of a compound from dimethyl-L-tartrate and benzophenone, shedding light on the conformation and interactions within the molecule (Irurre et al., 1992).
Molecular Structure Analysis
Molecular structure analysis, including vibrational circular dichroism (VCD) studies, provides insights into the structural characteristics and intramolecular hydrogen bonding of compounds like (R,S)-2,2-Dimethyl-1,3-dioxolane-4-methanol-d5 (Nakao, Kyōgoku, & Sugeta, 1986). These analyses contribute significantly to understanding the molecular conformation and the impact of hydrogen bonding on the compound's properties.
Chemical Reactions and Properties
Research on similar compounds has focused on bidentate chelation-controlled asymmetric synthesis, demonstrating the versatility and reactivity of these molecules in creating α-hydroxy esters (Jung, Ho, & Kim, 2000). These studies underscore the compound's potential in synthetic organic chemistry, particularly in asymmetric synthesis.
Physical Properties Analysis
The physical properties of compounds akin to (R,S)-2,2-Dimethyl-1,3-dioxolane-4-methanol-d5, such as crystal structure and solvation effects, have been the subject of scientific investigation. For instance, the study of crystalline inclusion compounds reveals the kinetics of desolvation and structural details, providing valuable information on the stability and behavior of these molecules under different conditions (Bourne, Oom, & Toda, 1997).
Chemical Properties Analysis
The chemical properties of similar dioxolane compounds have been explored through various reactions, including the resolution of isomers via enzymatic processes, highlighting the enantioselectivity and potential for specific synthetic applications (Miyazawa et al., 1999). This aspect is crucial for understanding the compound's reactivity and potential for producing enantiomerically pure substances.
Scientific Research Applications
Asymmetric Synthesis
(R,S)-2,2-Dimethyl-1,3-dioxolane-4-methanol-d5 has been explored as a chiral auxiliary for asymmetric synthesis, particularly in the context of α-hydroxy esters. Its utilization in bidentate chelation-controlled alkylation of glycolate enolate has been demonstrated to produce enantioselective outcomes, showcasing its potential in stereoselective chemical transformations (Jung et al., 2000).
Heterogeneously Catalyzed Condensations
The compound has been implicated in studies focusing on heterogeneously catalyzed condensations, particularly involving glycerol with various aldehydes and ketones. These studies explore its role in the formation of cyclic acetals and ketals, potentially offering novel pathways for the synthesis of platform chemicals derived from renewable materials (Deutsch et al., 2007).
Synthesis of Diol Derivatives
Efforts have also been made to synthesize diol derivatives from (R,S)-2,2-Dimethyl-1,3-dioxolane-4-methanol-d5, highlighting its versatility as a precursor in organic synthesis. The transformation of this compound into various diol configurations has been achieved with high yields, demonstrating its utility in the synthesis of complex organic molecules (Leftheris & Goodman, 1989).
Enantiomerically Pure Compounds
Research has also been conducted on the preparation of enantiomerically pure compounds using (R,S)-2,2-Dimethyl-1,3-dioxolane-4-methanol-d5 as a starting material. These studies contribute to the field of chiral chemistry, providing insights into the synthesis of pure enantiomers for applications in asymmetric catalysis and synthesis (Casati et al., 2011).
Vibrational Circular Dichroism Studies
The compound has been subject to vibrational circular dichroism (VCD) studies, particularly focusing on the OH stretching vibration. These studies provide valuable information on the intramolecular hydrogen bonding characteristics and conformations of the molecule, contributing to the understanding of its stereochemical properties (Nakao et al., 1986).
Mechanism of Action
Safety and Hazards
properties
IUPAC Name |
dideuterio-(4,5,5-trideuterio-2,2-dimethyl-1,3-dioxolan-4-yl)methanol | |
---|---|---|
Source | PubChem | |
URL | https://pubchem.ncbi.nlm.nih.gov | |
Description | Data deposited in or computed by PubChem | |
InChI |
InChI=1S/C6H12O3/c1-6(2)8-4-5(3-7)9-6/h5,7H,3-4H2,1-2H3/i3D2,4D2,5D | |
Source | PubChem | |
URL | https://pubchem.ncbi.nlm.nih.gov | |
Description | Data deposited in or computed by PubChem | |
InChI Key |
RNVYQYLELCKWAN-ZDGANNJSSA-N | |
Source | PubChem | |
URL | https://pubchem.ncbi.nlm.nih.gov | |
Description | Data deposited in or computed by PubChem | |
Canonical SMILES |
CC1(OCC(O1)CO)C | |
Source | PubChem | |
URL | https://pubchem.ncbi.nlm.nih.gov | |
Description | Data deposited in or computed by PubChem | |
Isomeric SMILES |
[2H]C1(C(OC(O1)(C)C)([2H])C([2H])([2H])O)[2H] | |
Source | PubChem | |
URL | https://pubchem.ncbi.nlm.nih.gov | |
Description | Data deposited in or computed by PubChem | |
Molecular Formula |
C6H12O3 | |
Source | PubChem | |
URL | https://pubchem.ncbi.nlm.nih.gov | |
Description | Data deposited in or computed by PubChem | |
Molecular Weight |
137.19 g/mol | |
Source | PubChem | |
URL | https://pubchem.ncbi.nlm.nih.gov | |
Description | Data deposited in or computed by PubChem | |
Product Name |
(R,S)-2,2-Dimethyl-1,3-dioxolane-4-methanol-d5 |
Synthesis routes and methods I
Procedure details
Synthesis routes and methods II
Procedure details
Synthesis routes and methods III
Procedure details
Synthesis routes and methods IV
Procedure details
Synthesis routes and methods V
Procedure details
Disclaimer and Information on In-Vitro Research Products
Please be aware that all articles and product information presented on BenchChem are intended solely for informational purposes. The products available for purchase on BenchChem are specifically designed for in-vitro studies, which are conducted outside of living organisms. In-vitro studies, derived from the Latin term "in glass," involve experiments performed in controlled laboratory settings using cells or tissues. It is important to note that these products are not categorized as medicines or drugs, and they have not received approval from the FDA for the prevention, treatment, or cure of any medical condition, ailment, or disease. We must emphasize that any form of bodily introduction of these products into humans or animals is strictly prohibited by law. It is essential to adhere to these guidelines to ensure compliance with legal and ethical standards in research and experimentation.