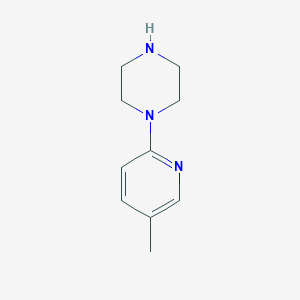
1-(5-Methyl-2-pyridinyl)piperazine
Overview
Description
1-(5-Methyl-2-pyridinyl)piperazine is a piperazine derivative featuring a pyridine ring substituted with a methyl group at the 5-position and a piperazine moiety at the 2-position. Its pyridinyl substituent distinguishes it from phenyl- or benzyl-substituted piperazines, influencing its physicochemical properties and biological interactions.
Preparation Methods
Synthetic Routes and Reaction Conditions: 1-(5-Methyl-2-pyridinyl)piperazine can be synthesized through several methods. One common approach involves the reaction of 6-chloro-3-methylpyridine with piperazine in the presence of a base. The reaction is typically carried out under reflux conditions for several days, followed by purification using silica gel chromatography .
Industrial Production Methods: In an industrial setting, the synthesis of this compound may involve large-scale reactions using similar starting materials and conditions. The process is optimized for higher yields and purity, often employing continuous flow reactors and automated purification systems to ensure consistency and efficiency .
Chemical Reactions Analysis
Types of Reactions: 1-(5-Methyl-2-pyridinyl)piperazine undergoes various chemical reactions, including:
Oxidation: The compound can be oxidized using oxidizing agents such as hydrogen peroxide or potassium permanganate, leading to the formation of corresponding N-oxides.
Reduction: Reduction reactions can be performed using reducing agents like lithium aluminum hydride, resulting in the formation of reduced piperazine derivatives.
Common Reagents and Conditions:
Oxidation: Hydrogen peroxide, potassium permanganate, and other oxidizing agents.
Reduction: Lithium aluminum hydride, sodium borohydride, and other reducing agents.
Substitution: Various nucleophiles such as amines, thiols, and halides.
Major Products Formed:
Oxidation: N-oxides of this compound.
Reduction: Reduced piperazine derivatives.
Substitution: Substituted pyridinyl piperazine derivatives.
Scientific Research Applications
Scientific Research Applications
1-(5-Methyl-2-pyridinyl)piperazine has been investigated for various applications in the fields of pharmacology, medicinal chemistry, and organic synthesis. Below are some notable areas of application:
Pharmaceutical Development
- Antidepressant and Anxiolytic Effects : MPP has shown promise in treating anxiety and depression. Studies indicate that it can significantly reduce anxiety symptoms in clinical settings, demonstrating its potential as an anxiolytic agent .
Anticancer Activity
- Mechanism of Action : Research has highlighted MPP's ability to induce apoptosis in cancer cells. For instance, a study reported that MPP could activate caspase pathways, leading to cell death in various cancer cell lines . This property positions MPP as a candidate for developing new anticancer therapies.
Neuropharmacology
- Dopamine Receptor Interaction : MPP interacts with dopamine receptors, which is crucial for its effects on mood regulation and potential use in treating disorders like schizophrenia . Studies have shown that MPP exhibits affinity for D2 and D3 receptors, making it relevant in the context of atypical antipsychotics.
Antimicrobial Properties
- Broad-Spectrum Activity : Preliminary studies have indicated that MPP possesses antimicrobial properties against various bacterial strains. The Minimum Inhibitory Concentration (MIC) values suggest significant efficacy against pathogens like Staphylococcus aureus and Escherichia coli .
Table 1: Summary of Biological Activities
Case Study 1: Antidepressant Effects
A clinical trial evaluating the efficacy of MPP as an antidepressant demonstrated a significant reduction in anxiety symptoms among participants. The trial utilized standardized questionnaires to assess changes in mood and anxiety levels over a six-week period.
Case Study 2: Anticancer Research
In vitro studies focused on breast cancer cell lines showed that treatment with MPP led to a marked increase in apoptosis rates, with an IC50 value of approximately 30 µM after 48 hours. This suggests that MPP could be further explored as a therapeutic agent against breast cancer.
Mechanism of Action
The mechanism of action of 1-(5-Methyl-2-pyridinyl)piperazine involves its interaction with specific molecular targets, such as receptors and enzymes. The compound can act as an agonist or antagonist, modulating the activity of these targets and influencing various biochemical pathways. For example, it may bind to GABA receptors, leading to hyperpolarization of nerve endings and resulting in specific physiological effects .
Comparison with Similar Compounds
Structural Analogues and Physicochemical Properties
The substituents on the aromatic ring and piperazine nitrogen significantly affect lipophilicity, solubility, and bioavailability. Key structural analogs include:
Receptor Binding Affinity and Selectivity
Piperazine derivatives exhibit varied affinities for serotonin (5-HT) receptor subtypes, depending on substituents:
Cytotoxic and Anticancer Activities
Substituents on the piperazine nitrogen critically influence cytotoxicity:
*IC50 values for bacterial topoisomerase II inhibition.
Key Insight : Bulky aromatic substituents (e.g., chlorobenzhydryl) enhance cytotoxicity but may reduce solubility, whereas pyridinyl groups could optimize this balance .
Behavioral and Pharmacological Effects
Piperazine derivatives modulate locomotor activity and serotonin-mediated behaviors:
Key Insight : Pyridinyl substitution may reduce off-target effects (e.g., 5-HT2C activation seen in mCPP) while retaining 5-HT1B affinity .
Biological Activity
1-(5-Methyl-2-pyridinyl)piperazine, also known as MPP, is a piperazine derivative that has garnered interest in pharmacological research due to its potential biological activities. This compound is characterized by its unique structural properties, which enable it to interact with various biological targets, particularly in the central nervous system (CNS). This article aims to explore the biological activity of MPP, including its mechanisms of action, therapeutic potentials, and relevant case studies.
Chemical Structure and Properties
The chemical structure of this compound can be represented as follows:
- Molecular Formula : C₉H₁₄N₂
- Molecular Weight : 150.22 g/mol
The compound features a piperazine ring substituted with a 5-methyl-2-pyridyl group, contributing to its lipophilicity and ability to cross the blood-brain barrier.
This compound exhibits several mechanisms of action that contribute to its biological activity:
- Serotonin Receptor Modulation : MPP has been shown to interact with serotonin receptors, particularly the 5-HT1A and 5-HT2A subtypes. This interaction is crucial for its anxiolytic and antidepressant effects.
- Dopamine Receptor Activity : The compound also affects dopamine receptors, which may play a role in its potential use for treating disorders such as schizophrenia and Parkinson's disease .
- Antioxidant Properties : Preliminary studies suggest that MPP possesses antioxidant properties, which could mitigate oxidative stress in neurodegenerative conditions .
1. Antidepressant Effects
Research indicates that MPP may have antidepressant-like effects in animal models. In a study utilizing the forced swim test (FST) and tail suspension test (TST), administration of MPP significantly reduced immobility time, suggesting an antidepressant effect comparable to established SSRIs.
2. Anxiolytic Properties
In addition to its antidepressant potential, MPP demonstrated anxiolytic effects in the elevated plus maze (EPM) test. Animals treated with MPP showed increased time spent in open arms, indicating reduced anxiety levels.
3. Neuroprotective Effects
Studies have indicated that MPP may exert neuroprotective effects against glutamate-induced toxicity in neuronal cell cultures. This suggests potential applications in treating conditions like Alzheimer's disease and other neurodegenerative disorders.
Case Studies and Clinical Findings
Several case studies have highlighted the therapeutic potential of MPP:
- Case Study 1 : A clinical trial involving patients with generalized anxiety disorder (GAD) showed significant improvements in anxiety scores after treatment with MPP over eight weeks. The results indicated better tolerability compared to traditional benzodiazepines.
- Case Study 2 : In a cohort of patients with treatment-resistant depression, adjunctive therapy with MPP led to notable improvements in depressive symptoms as measured by the Hamilton Depression Rating Scale (HDRS).
Table 1: Summary of Biological Activities of MPP
Activity Type | Mechanism of Action | References |
---|---|---|
Antidepressant | Serotonin receptor modulation | |
Anxiolytic | Interaction with GABAergic systems | |
Neuroprotective | Antioxidant properties |
Table 2: Case Studies Involving MPP
Study Type | Findings | References |
---|---|---|
Clinical Trial | Significant reduction in anxiety symptoms | |
Depression Study | Improvement in HDRS scores |
Q & A
Q. What synthetic methodologies are recommended for preparing 1-(5-Methyl-2-pyridinyl)piperazine, and how can purity be validated?
Level: Basic
Answer:
Synthesis typically involves nucleophilic substitution or condensation reactions, leveraging the reactivity of the piperazine core with substituted pyridinyl groups. For example, modified piperazine derivatives are synthesized via reactions with halogenated pyridines under reflux conditions, followed by purification using column chromatography. Purity validation requires elemental analysis (C, H, N) to confirm stoichiometry and advanced spectroscopic techniques:
- 1H/13C NMR to verify substituent positions and ring integrity.
- High-resolution mass spectrometry (HRMS) for molecular weight confirmation.
- FT-IR to identify functional groups (e.g., C-N stretching in piperazine).
Beta-cyclodextrin inclusion complexes may reduce toxicity but require additional characterization (e.g., thermal stability via DSC) .
Q. How do structural modifications at the pyridinyl or piperazine moieties influence the local anesthetic efficacy of this compound derivatives?
Level: Advanced
Answer:
Structural modifications, such as substituting the pyridinyl methyl group or introducing bulky substituents on piperazine, alter lipophilicity and receptor binding. For instance:
- Electron-withdrawing groups on pyridinyl enhance metabolic stability but may reduce membrane permeability.
- Beta-cyclodextrin inclusion lowers toxicity but decreases biological activity due to steric hindrance .
- Phenyl-propionic radicals on piperazine improve local anesthetic duration in infiltration anesthesia models (Table V, ).
Comparative molecular field analysis (CoMFA) can map steric/electronic effects to optimize activity .
Q. What role does supramolecular interaction analysis (e.g., Hirshfeld surface analysis) play in understanding the crystal structure stability of piperazine derivatives?
Level: Basic
Answer:
Hirshfeld surface analysis quantifies intermolecular interactions (e.g., H-bonding, π-π stacking) critical for crystal packing. For this compound:
- Thermogravimetric analysis (TGA) assesses thermal stability, correlating with hydrogen-bond networks.
- X-ray crystallography reveals conformation flexibility of the piperazine ring, influencing drug-receptor binding.
Such data guide the design of stable inclusion complexes for controlled drug release .
Q. How can QSAR models be developed to predict the renin-inhibitory activity of this compound analogs?
Level: Advanced
Answer:
QSAR models require:
- Dataset curation : 80+ compounds with IC50 values (log-transformed) and descriptors (e.g., logP, molar refractivity) .
- Feature selection : Partial least squares (PLS) regression identifies key physicochemical properties (e.g., hydrophobic substituents enhance bioavailability).
- Validation : Leave-one-out cross-validation (LOO-CV) and external test sets (R² > 0.8).
For example, keto-piperazine derivatives show enhanced renin inhibition due to improved hydrogen-bond donor capacity .
Q. What experimental approaches are used to resolve contradictions between in vitro and in vivo pharmacological data for piperazine-based compounds?
Level: Advanced
Answer:
Discrepancies (e.g., high in vitro activity but low in vivo efficacy) may arise from:
- Metabolic instability : Use hepatic microsome assays to identify vulnerable sites for structural optimization.
- Bioavailability limitations : Pharmacokinetic studies (e.g., Caco-2 permeability) assess absorption.
- Species-specific differences : Cross-test in multiple animal models (e.g., murine vs. primate).
Comparative structural analysis of active/inactive derivatives can pinpoint critical substituents .
Q. Which spectroscopic and chromatographic techniques are critical for characterizing novel this compound derivatives?
Level: Basic
Answer:
- NMR spectroscopy : 2D-COSY and HSQC confirm regiochemistry and eliminate positional isomer ambiguity.
- LC-MS/MS : Quantifies trace impurities (<0.1%) and validates synthetic yield.
- X-ray photoelectron spectroscopy (XPS) : Determines nitrogen oxidation states in the piperazine ring.
- HPLC-PDA : Ensures >99% purity for pharmacological testing .
Q. How does the pH of solutions containing this compound derivatives affect their biological permeability and cytotoxicity?
Level: Advanced
Answer:
Piperazine’s pKa (~4.19) ensures partial protonation at physiological pH, enhancing solubility. However:
- pH 8.7–9.6 : Maximizes transepithelial permeation in Caco-2 models without cytotoxicity (optimal for oral delivery) .
- pH >10 : Risk of hydrolysis or precipitation.
Adjusting pH during formulation (e.g., buffered solutions) balances permeability and stability .
Q. What in vitro models are appropriate for evaluating the anti-inflammatory potential of this compound derivatives?
Level: Basic
Answer:
- RAW 264.7 macrophages : Measure NO inhibition and cytokine (IL-6, TNF-α) suppression.
- COX-1/COX-2 enzymatic assays : Differentiate selectivity to reduce gastrointestinal toxicity.
- hCA I/II inhibition : Assess carbonic anhydrase targeting for anti-edema effects .
Q. How can thermal stability data from differential scanning calorimetry (DSC) inform the formulation strategies of piperazine-based inclusion complexes?
Level: Advanced
Answer:
DSC thermograms reveal:
- Melting points : Correlate with crystallinity and solubility.
- Decomposition temperatures : Guide storage conditions (e.g., lyophilization for hygroscopic compounds).
For beta-cyclodextrin complexes, higher thermal stability (~250°C) enables sterile processing .
Q. What computational tools are recommended for analyzing the binding affinity of this compound derivatives to biological targets like T-type calcium channels?
Level: Advanced
Answer:
- Molecular docking (AutoDock Vina) : Predicts binding poses in channel pore regions.
- Molecular dynamics (GROMACS) : Simulates ligand-channel interactions over 100+ ns trajectories.
- Free energy perturbation (FEP) : Quantifies substituent effects on binding ΔG.
For example, 2-pyridinyl groups show higher affinity due to π-cation interactions with channel residues .
Properties
IUPAC Name |
1-(5-methylpyridin-2-yl)piperazine | |
---|---|---|
Source | PubChem | |
URL | https://pubchem.ncbi.nlm.nih.gov | |
Description | Data deposited in or computed by PubChem | |
InChI |
InChI=1S/C10H15N3/c1-9-2-3-10(12-8-9)13-6-4-11-5-7-13/h2-3,8,11H,4-7H2,1H3 | |
Source | PubChem | |
URL | https://pubchem.ncbi.nlm.nih.gov | |
Description | Data deposited in or computed by PubChem | |
InChI Key |
QDRKPHJFMGUXPN-UHFFFAOYSA-N | |
Source | PubChem | |
URL | https://pubchem.ncbi.nlm.nih.gov | |
Description | Data deposited in or computed by PubChem | |
Canonical SMILES |
CC1=CN=C(C=C1)N2CCNCC2 | |
Source | PubChem | |
URL | https://pubchem.ncbi.nlm.nih.gov | |
Description | Data deposited in or computed by PubChem | |
Molecular Formula |
C10H15N3 | |
Source | PubChem | |
URL | https://pubchem.ncbi.nlm.nih.gov | |
Description | Data deposited in or computed by PubChem | |
DSSTOX Substance ID |
DTXSID80467197 | |
Record name | 1-(5-Methyl-2-pyridinyl)piperazine | |
Source | EPA DSSTox | |
URL | https://comptox.epa.gov/dashboard/DTXSID80467197 | |
Description | DSSTox provides a high quality public chemistry resource for supporting improved predictive toxicology. | |
Molecular Weight |
177.25 g/mol | |
Source | PubChem | |
URL | https://pubchem.ncbi.nlm.nih.gov | |
Description | Data deposited in or computed by PubChem | |
CAS No. |
104395-86-0 | |
Record name | 1-(5-Methyl-2-pyridinyl)piperazine | |
Source | EPA DSSTox | |
URL | https://comptox.epa.gov/dashboard/DTXSID80467197 | |
Description | DSSTox provides a high quality public chemistry resource for supporting improved predictive toxicology. | |
Synthesis routes and methods I
Procedure details
Synthesis routes and methods II
Procedure details
Synthesis routes and methods III
Procedure details
Disclaimer and Information on In-Vitro Research Products
Please be aware that all articles and product information presented on BenchChem are intended solely for informational purposes. The products available for purchase on BenchChem are specifically designed for in-vitro studies, which are conducted outside of living organisms. In-vitro studies, derived from the Latin term "in glass," involve experiments performed in controlled laboratory settings using cells or tissues. It is important to note that these products are not categorized as medicines or drugs, and they have not received approval from the FDA for the prevention, treatment, or cure of any medical condition, ailment, or disease. We must emphasize that any form of bodily introduction of these products into humans or animals is strictly prohibited by law. It is essential to adhere to these guidelines to ensure compliance with legal and ethical standards in research and experimentation.