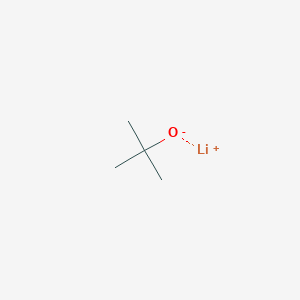
Lithium tert-butoxide
Overview
Description
Lithium tert-butoxide is an organolithium compound with the chemical formula LiOC(CH₃)₃. It appears as a white solid and is widely used as a strong base in organic synthesis. This compound is often depicted as a salt, and it behaves as such in many reactions, although it is not ionized in solution .
Mechanism of Action
Target of Action
Lithium tert-butoxide is a metalorganic compound that primarily targets organic compounds in chemical reactions . It is often used as a strong base in organic synthesis .
Mode of Action
As a strong base, this compound is easily protonated . It is used to prepare other tert-butoxide compounds such as copper (I) t-butoxide and hexa (tert-butoxy)dimolybdenum (III) . It is also used to promote the α-alkylation reaction of ketones with primary alcohols, without the addition of any transition metal catalyst .
Biochemical Pathways
this compound is involved in various biochemical pathways. It is used in the synthesis of carbamates and their derivatives, which play a pivotal role in bioactive compounds . It can also be used to deprotonate 1-(phenylseleno) alkenes and bis(phenylseleno) acetals .
Pharmacokinetics
It is known that it is often generated in situ for laboratory use because samples are sensitive and older samples are often of poor quality .
Result of Action
The result of this compound’s action is the production of new compounds through its role as a strong base in organic synthesis . For example, it can promote the α-alkylation reaction of ketones with primary alcohols, leading to the formation of new compounds .
Action Environment
this compound is sensitive to environmental conditions. It should be kept away from incompatible materials, light, ignition sources, excess heat, exposure to moist air or water, and confined spaces . It is also known to react violently with water and its containers may explode when heated .
Biochemical Analysis
Biochemical Properties
Lithium tert-butoxide is a weakly basic and nucleophilic alkali metal oxide . It is commonly used as an initiator for anionic polymerization . In combination with potassium diisopropylamide, this compound can be used to deprotonate 1-(phenylseleno) alkenes and bis(phenylseleno) acetals .
Cellular Effects
It is known that it can promote the α-alkylation reaction of ketones with primary alcohols, without the addition of any transition metal catalyst .
Molecular Mechanism
As a strong base, this compound is easily protonated . It is used to prepare other tert-butoxide compounds such as copper (I) t-butoxide and hexa(tert-butoxy)dimolybdenum (III) .
Temporal Effects in Laboratory Settings
This compound is commercially available as a solution and as a solid, but it is often generated in situ for laboratory use because samples are so sensitive and older samples are often of poor quality . It can be obtained by treating tert-butanol with butyl lithium .
Metabolic Pathways
This compound is known to efficiently promote the α-alkylation reaction of ketones with primary alcohols
Transport and Distribution
It is known that this compound is a mild base used in organic synthesis .
Subcellular Localization
It is known that this compound is a mild base used in organic synthesis .
Preparation Methods
Synthetic Routes and Reaction Conditions: Lithium tert-butoxide can be synthesized by treating tert-butanol with butyl lithium. The reaction is typically carried out under an inert atmosphere to prevent moisture and air from affecting the reaction . The general reaction is as follows:
C4H9OH+LiC4H9→LiOC(CH3)3+C4H10
Industrial Production Methods: In industrial settings, this compound is often generated in situ due to its sensitivity and the poor quality of older samples. A common method involves reacting lithium metal with tert-butanol in a solvent such as tetrahydrofuran under an inert atmosphere at elevated temperatures .
Types of Reactions:
Oxidation: this compound does not typically undergo oxidation reactions due to its strong basic nature.
Reduction: It is involved in the reduction of ketones to secondary alcohols.
Substitution: It can deprotonate various substrates, facilitating substitution reactions.
Common Reagents and Conditions:
Reagents: Common reagents include primary alcohols, ketones, and various organic substrates.
Conditions: Reactions are typically carried out under an inert atmosphere to prevent moisture and air from affecting the reaction.
Major Products:
Reduction Products: Secondary alcohols from the reduction of ketones.
Substitution Products: Various substituted organic compounds depending on the substrate used
Scientific Research Applications
Lithium tert-butoxide has a wide range of applications in scientific research:
Chemistry: It is used as an initiator for anionic polymerization and as a base in various organic synthesis reactions.
Biology: While not directly used in biological systems, it plays a role in the synthesis of biologically active compounds.
Medicine: It is used in the preparation of pharmaceutical intermediates.
Comparison with Similar Compounds
- Sodium tert-butoxide
- Potassium tert-butoxide
- Lithium isopropoxide
Comparison:
- Basicity: Lithium tert-butoxide is a strong base, similar to sodium and potassium tert-butoxide. its basicity is slightly lower than that of potassium tert-butoxide due to the smaller size of the lithium ion.
- Reactivity: It is more reactive than sodium tert-butoxide due to the smaller ionic radius of lithium, which allows for closer interaction with the substrate.
- Solubility: this compound is more soluble in organic solvents compared to its sodium and potassium counterparts .
This compound stands out due to its unique combination of strong basicity, high reactivity, and good solubility in organic solvents, making it a valuable reagent in organic synthesis.
Properties
CAS No. |
1907-33-1 |
---|---|
Molecular Formula |
C4H10LiO |
Molecular Weight |
81.1 g/mol |
IUPAC Name |
lithium;2-methylpropan-2-olate |
InChI |
InChI=1S/C4H10O.Li/c1-4(2,3)5;/h5H,1-3H3; |
InChI Key |
UOHBMRODJBFDPN-UHFFFAOYSA-N |
SMILES |
[Li+].CC(C)(C)[O-] |
Isomeric SMILES |
[Li+].CC(C)(C)[O-] |
Canonical SMILES |
[Li].CC(C)(C)O |
Key on ui other cas no. |
1907-33-1 |
Pictograms |
Flammable; Corrosive; Irritant |
Synonyms |
2-Methyl-2-propanol Lithium Salt; Lithium t-butoxide; Lithium tert-Butanolate; _x000B_Lithium tert-Butylate; tert-Butanol Lithium Salt; tert-Butoxylithium; |
Origin of Product |
United States |
Synthesis routes and methods I
Procedure details
Synthesis routes and methods II
Procedure details
Retrosynthesis Analysis
AI-Powered Synthesis Planning: Our tool employs the Template_relevance Pistachio, Template_relevance Bkms_metabolic, Template_relevance Pistachio_ringbreaker, Template_relevance Reaxys, Template_relevance Reaxys_biocatalysis model, leveraging a vast database of chemical reactions to predict feasible synthetic routes.
One-Step Synthesis Focus: Specifically designed for one-step synthesis, it provides concise and direct routes for your target compounds, streamlining the synthesis process.
Accurate Predictions: Utilizing the extensive PISTACHIO, BKMS_METABOLIC, PISTACHIO_RINGBREAKER, REAXYS, REAXYS_BIOCATALYSIS database, our tool offers high-accuracy predictions, reflecting the latest in chemical research and data.
Strategy Settings
Precursor scoring | Relevance Heuristic |
---|---|
Min. plausibility | 0.01 |
Model | Template_relevance |
Template Set | Pistachio/Bkms_metabolic/Pistachio_ringbreaker/Reaxys/Reaxys_biocatalysis |
Top-N result to add to graph | 6 |
Feasible Synthetic Routes
Q1: How does lithium tert-butoxide interact with ketones?
A1: this compound can react with ketones through two main pathways: addition and reduction. The addition pathway leads to the formation of tertiary alcohols, while the reduction pathway yields secondary alcohols. The ratio of these pathways can be influenced by the specific reaction conditions, including the presence of salts []. For instance, adding salts like potassium methoxide or lithium methoxide to dialkylmagnesium compounds prior to reaction with ketones can significantly reduce the extent of reduction [].
Q2: How does this compound interact with acrylamide in asymmetric Michael reactions?
A2: this compound plays a dual role in this reaction. Firstly, it deprotonates the ketone, forming a lithium enolate. Secondly, it interacts with the chiral lithium binaphtholate catalyst, influencing the enolate's orientation and promoting enantioselective carbon-carbon bond formation with the acrylamide [].
Q3: What is the role of this compound in the synthesis of bis(3-indolyl)methanes (BIMs)?
A3: this compound acts as a base in the alkylation reaction of indoles with alcohols, leading to the formation of BIMs. Interestingly, air acts as an oxidant in this transformation, and the presence of the amide group in the indole substrate is crucial for activating the alkyne towards nucleophilic attack [].
Q4: What is the molecular formula and weight of this compound?
A4: The molecular formula of Lithium 2-methylpropan-2-olate is C4H9LiO, and its molecular weight is 80.05 g/mol.
Q5: What spectroscopic techniques are useful for characterizing this compound and its derivatives?
A5: Several techniques are valuable, including:* NMR Spectroscopy (1H, 6Li, 7Li, 13C): Provides information about the structure, aggregation state, and dynamics of this compound and its complexes [, , ]. * IR Spectroscopy: Useful for identifying functional groups and studying interactions between this compound and other species [].
Q6: What is the thermal stability of this compound?
A6: this compound decomposes at temperatures above -30°C []. For optimal results, it is crucial to handle and store the reagent at low temperatures (≤ -50°C) to prevent decomposition.
Q7: How does the presence of this compound affect the stability of tert-butyllithium?
A7: When combined, they form mixed aggregates that exhibit enhanced stability compared to tert-butyllithium alone. This increased stability is attributed to the formation of larger aggregates with reduced reactivity [].
Q8: How does the solvent influence the stability and reactivity of this compound?
A8: The solvent plays a significant role in the aggregation state and reactivity of this compound. For instance, it forms different aggregates with methyl α-lithioisobutyrate in THF depending on the this compound concentration []. Furthermore, the use of diglyme as a solvent was found to be crucial in achieving high C/O regioselectivity in the monofluoromethylation of β-ketoesters with fluoromethyl iodide in the presence of this compound [].
Q9: What type of reactions can this compound catalyze?
A9: this compound is a strong base and can catalyze a wide variety of reactions, including:* Polymerization Reactions: Used as an initiator or modifier in anionic polymerization of (meth)acrylates and other monomers [, , , , , , , ].* Addition Reactions: Catalyzes the addition of carbon disulfide to epoxides and thiiranes to synthesize di- and trithiocarbonates [].* Arylation Reactions: Promotes direct arylation of electron-rich heterocycles with aryl halides [, ].* Carboxylation Reactions: Facilitates the carboxylation of unprotected indoles and pyrroles with carbon dioxide [].* C-H Amination Reactions: Enables C(sp3)-H amination of amides using N-haloimides under visible light irradiation [].* Aldol Reactions: Can be used as a base in silver-catalyzed direct aldol reactions of benzophenone glycine imines with aldehydes [].
Q10: How does the structure of the lithium alkoxide influence its catalytic activity in interesterification reactions?
A10: The structure of the alkyl chain significantly influences the alkoxide's solubility and, consequently, its catalytic activity. Branched alkoxides like this compound exhibit higher activity in interesterification reactions compared to linear alkoxides due to their increased solubility [].
Q11: How does this compound influence the regioselectivity in reactions?
A11: In the direct arylation of indolizine, this compound promotes selective arylation at position 5, contrasting the selectivity observed in palladium-catalyzed reactions []. Similarly, it facilitates the regioselective α-amination of ethers using N-chloroimides, leading to the formation of hemiaminal ethers []. In the monofluoromethylation of β-ketoesters with fluoromethyl iodide, using this compound as the base significantly favors carbon-selective monofluoromethylation over oxygen-selective reactions [].
Q12: How has computational chemistry been used to study this compound systems?
A12: Computational methods like MNDO (Modified Neglect of Differential Overlap) have been used to model and predict the structures of this compound aggregates []. These calculations provided insights into the stability and geometry of different aggregates, revealing a tendency to form cubical (O-Li)4 cores. In the context of C-H amination reactions, computations were used to investigate the formation of a halogen-bonded intermediate between this compound and N-haloimides, supporting the proposed mechanism involving this complex [].
Q13: How does the counterion influence the reactivity of tert-butoxide in anionic polymerization?
A13: The size of the counterion (Li+, Na+, K+) significantly influences the polymerization rate. For N-phenylitaconimide, smaller counterions result in faster polymerization, opposite to the trend observed with N-phenylmaleimide []. This difference highlights the importance of monomer structure in determining counterion effects on reactivity.
Q14: How does the structure of the lithium alkoxide affect its activity in interesterification reactions?
A14: The alkyl chain structure of the lithium alkoxide directly impacts its solubility and catalytic activity. Branched alkoxides, like this compound, exhibit enhanced solubility and higher activity compared to linear counterparts in the interesterification of rapeseed oil with methyl acetate [].
Q15: How can the stability of this compound solutions be improved?
A15: To prevent decomposition, it's crucial to:* Use high-purity reagents: Ensure the copper(I) salts, solvents, and alkyllithium solutions are free from impurities like alkoxides or hydroxides, which can catalyze decomposition [].* Maintain low temperatures: Store and handle the reagent at temperatures below -50°C to avoid thermal decomposition [].* Use an inert atmosphere: Handle this compound under an inert atmosphere (e.g., nitrogen or argon) to prevent reaction with moisture and oxygen, which can lead to degradation.
Q16: What safety precautions should be taken when handling this compound?
A16: this compound is a strong base and can cause burns. It is also flammable and reacts violently with water. Therefore, it is essential to:* Handle with care: Always wear appropriate personal protective equipment, including gloves, goggles, and a lab coat.* Work in a well-ventilated area: Use a fume hood to prevent inhalation of fumes.* Keep away from ignition sources: Avoid contact with heat, sparks, and open flames.* Store properly: Store in a cool, dry place away from incompatible materials.* Dispose of properly: Follow local regulations for the safe disposal of this compound and related waste.
Q17: What are the applications of this compound in materials science?
A17: * Solid-State Electrolytes: this compound is a precursor for atomic layer deposition (ALD) of lithium-containing thin films, such as lithium phosphorus oxynitride (LiPON) [, ], lithium aluminum oxide (LiAlOx) [, ], and lithium carbonate (Li2CO3) []. These materials are promising solid-state electrolytes for all-solid-state batteries, offering improved safety and energy density compared to conventional liquid electrolytes.* Electro-spun Membranes: Used in the preparation of EVOH-SO3Li/SiO2 non-woven membranes via electrospinning for potential applications in lithium-ion batteries [].
Q18: Can this compound be used to synthesize nanomaterials?
A18: Yes, it has been employed in the synthesis of gold nanorods and nanowires. The morphology of the resulting nanostructures can be controlled by adjusting the ratio of gold halide to this compound and the presence of additives like AuCl, AuI, and LiBr [].
Q19: Are there alternatives to this compound in specific reactions?
A19: Yes, the choice of an alternative base depends on the specific reaction and desired outcome. Some possible substitutes include:* Other Lithium Alkoxides: Lithium methoxide, lithium ethoxide, or lithium isopropoxide can be considered depending on the substrate and desired reactivity [, ].* Other Strong Bases: Potassium tert-butoxide, sodium hydride, or LDA (lithium diisopropylamide) might be suitable depending on the reaction conditions and pKa requirements.
Disclaimer and Information on In-Vitro Research Products
Please be aware that all articles and product information presented on BenchChem are intended solely for informational purposes. The products available for purchase on BenchChem are specifically designed for in-vitro studies, which are conducted outside of living organisms. In-vitro studies, derived from the Latin term "in glass," involve experiments performed in controlled laboratory settings using cells or tissues. It is important to note that these products are not categorized as medicines or drugs, and they have not received approval from the FDA for the prevention, treatment, or cure of any medical condition, ailment, or disease. We must emphasize that any form of bodily introduction of these products into humans or animals is strictly prohibited by law. It is essential to adhere to these guidelines to ensure compliance with legal and ethical standards in research and experimentation.