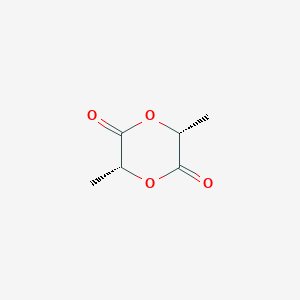
(3R,6R)-3,6-dimethyl-1,4-dioxane-2,5-dione
Overview
Description
(3R,6R)-3,6-Dimethyl-1,4-dioxane-2,5-dione is a chiral lactone compound with a six-membered ring structure. It is known for its applications in various fields, including organic synthesis and pharmaceutical research. The compound’s unique stereochemistry makes it an interesting subject for studies related to chirality and enantioselectivity.
Preparation Methods
Synthetic Routes and Reaction Conditions: The synthesis of (3R,6R)-3,6-dimethyl-1,4-dioxane-2,5-dione typically involves the cyclization of appropriate diols or hydroxy acids under acidic conditions. One common method is the acid-catalyzed cyclization of 3,6-dimethyl-1,4-dioxane-2,5-diol. The reaction is carried out in the presence of a strong acid such as sulfuric acid or hydrochloric acid, which facilitates the formation of the lactone ring.
Industrial Production Methods: Industrial production of this compound may involve continuous flow processes to ensure high yield and purity. The use of catalysts and optimized reaction conditions, such as temperature and pressure control, can enhance the efficiency of the production process. Additionally, purification techniques like distillation or crystallization are employed to obtain the final product with the desired enantiomeric purity.
Chemical Reactions Analysis
Types of Reactions: (3R,6R)-3,6-Dimethyl-1,4-dioxane-2,5-dione undergoes various chemical reactions, including:
Oxidation: The compound can be oxidized to form corresponding carboxylic acids or ketones.
Reduction: Reduction reactions can convert the lactone to diols or hydroxy acids.
Substitution: Nucleophilic substitution reactions can introduce different functional groups into the molecule.
Common Reagents and Conditions:
Oxidation: Common oxidizing agents include potassium permanganate and chromium trioxide.
Reduction: Reducing agents such as lithium aluminum hydride or sodium borohydride are used.
Substitution: Nucleophiles like amines or alcohols can be used under basic or acidic conditions.
Major Products Formed:
Oxidation: Carboxylic acids or ketones.
Reduction: Diols or hydroxy acids.
Substitution: Various substituted derivatives depending on the nucleophile used.
Scientific Research Applications
(3R,6R)-3,6-Dimethyl-1,4-dioxane-2,5-dione has several scientific research applications:
Chemistry: It is used as a chiral building block in the synthesis of complex organic molecules.
Biology: The compound’s stereochemistry is studied for its effects on biological systems and enzyme interactions.
Medicine: It serves as an intermediate in the synthesis of pharmaceutical compounds with potential therapeutic effects.
Industry: The compound is used in the production of polymers and other materials with specific properties.
Mechanism of Action
The mechanism of action of (3R,6R)-3,6-dimethyl-1,4-dioxane-2,5-dione involves its interaction with molecular targets such as enzymes or receptors. The compound’s chiral nature allows it to fit into specific active sites, influencing the activity of the target molecules. This interaction can lead to various biological effects, depending on the pathway involved.
Comparison with Similar Compounds
(3R,6R)-3-benzyl-6-isopropyl-4-methylmorpholine-2,5-dione: Another chiral lactone with antifungal activity.
(3R,3’R,6’R)-lutein: A carotenoid with similar stereochemistry used in eye health.
Uniqueness: (3R,6R)-3,6-Dimethyl-1,4-dioxane-2,5-dione is unique due to its specific ring structure and stereochemistry, which make it a valuable compound for studying chirality and enantioselective reactions. Its applications in various fields, from organic synthesis to pharmaceutical research, highlight its versatility and importance.
Properties
IUPAC Name |
(3R,6R)-3,6-dimethyl-1,4-dioxane-2,5-dione | |
---|---|---|
Source | PubChem | |
URL | https://pubchem.ncbi.nlm.nih.gov | |
Description | Data deposited in or computed by PubChem | |
InChI |
InChI=1S/C6H8O4/c1-3-5(7)10-4(2)6(8)9-3/h3-4H,1-2H3/t3-,4-/m1/s1 | |
Source | PubChem | |
URL | https://pubchem.ncbi.nlm.nih.gov | |
Description | Data deposited in or computed by PubChem | |
InChI Key |
JJTUDXZGHPGLLC-QWWZWVQMSA-N | |
Source | PubChem | |
URL | https://pubchem.ncbi.nlm.nih.gov | |
Description | Data deposited in or computed by PubChem | |
Canonical SMILES |
CC1C(=O)OC(C(=O)O1)C | |
Source | PubChem | |
URL | https://pubchem.ncbi.nlm.nih.gov | |
Description | Data deposited in or computed by PubChem | |
Isomeric SMILES |
C[C@@H]1C(=O)O[C@@H](C(=O)O1)C | |
Source | PubChem | |
URL | https://pubchem.ncbi.nlm.nih.gov | |
Description | Data deposited in or computed by PubChem | |
Molecular Formula |
C6H8O4 | |
Source | PubChem | |
URL | https://pubchem.ncbi.nlm.nih.gov | |
Description | Data deposited in or computed by PubChem | |
Related CAS |
25038-75-9, 1044507-68-7 | |
Record name | D-Lactide homopolymer | |
Source | CAS Common Chemistry | |
URL | https://commonchemistry.cas.org/detail?cas_rn=25038-75-9 | |
Description | CAS Common Chemistry is an open community resource for accessing chemical information. Nearly 500,000 chemical substances from CAS REGISTRY cover areas of community interest, including common and frequently regulated chemicals, and those relevant to high school and undergraduate chemistry classes. This chemical information, curated by our expert scientists, is provided in alignment with our mission as a division of the American Chemical Society. | |
Explanation | The data from CAS Common Chemistry is provided under a CC-BY-NC 4.0 license, unless otherwise stated. | |
Record name | 1,4-Dioxane-2,5-dione, 3,6-dimethyl-, (3R,6R)-rel-, homopolymer | |
Source | CAS Common Chemistry | |
URL | https://commonchemistry.cas.org/detail?cas_rn=1044507-68-7 | |
Description | CAS Common Chemistry is an open community resource for accessing chemical information. Nearly 500,000 chemical substances from CAS REGISTRY cover areas of community interest, including common and frequently regulated chemicals, and those relevant to high school and undergraduate chemistry classes. This chemical information, curated by our expert scientists, is provided in alignment with our mission as a division of the American Chemical Society. | |
Explanation | The data from CAS Common Chemistry is provided under a CC-BY-NC 4.0 license, unless otherwise stated. | |
DSSTOX Substance ID |
DTXSID10873989 | |
Record name | D,D-Dilactide | |
Source | EPA DSSTox | |
URL | https://comptox.epa.gov/dashboard/DTXSID10873989 | |
Description | DSSTox provides a high quality public chemistry resource for supporting improved predictive toxicology. | |
Molecular Weight |
144.12 g/mol | |
Source | PubChem | |
URL | https://pubchem.ncbi.nlm.nih.gov | |
Description | Data deposited in or computed by PubChem | |
CAS No. |
13076-17-0 | |
Record name | D-Lactide | |
Source | CAS Common Chemistry | |
URL | https://commonchemistry.cas.org/detail?cas_rn=13076-17-0 | |
Description | CAS Common Chemistry is an open community resource for accessing chemical information. Nearly 500,000 chemical substances from CAS REGISTRY cover areas of community interest, including common and frequently regulated chemicals, and those relevant to high school and undergraduate chemistry classes. This chemical information, curated by our expert scientists, is provided in alignment with our mission as a division of the American Chemical Society. | |
Explanation | The data from CAS Common Chemistry is provided under a CC-BY-NC 4.0 license, unless otherwise stated. | |
Record name | Lactide, D- | |
Source | ChemIDplus | |
URL | https://pubchem.ncbi.nlm.nih.gov/substance/?source=chemidplus&sourceid=0013076170 | |
Description | ChemIDplus is a free, web search system that provides access to the structure and nomenclature authority files used for the identification of chemical substances cited in National Library of Medicine (NLM) databases, including the TOXNET system. | |
Record name | 1,4-Dioxane-2,5-dione, 3,6-dimethyl-, (3R,6R)- | |
Source | EPA Chemicals under the TSCA | |
URL | https://www.epa.gov/chemicals-under-tsca | |
Description | EPA Chemicals under the Toxic Substances Control Act (TSCA) collection contains information on chemicals and their regulations under TSCA, including non-confidential content from the TSCA Chemical Substance Inventory and Chemical Data Reporting. | |
Record name | D,D-Dilactide | |
Source | EPA DSSTox | |
URL | https://comptox.epa.gov/dashboard/DTXSID10873989 | |
Description | DSSTox provides a high quality public chemistry resource for supporting improved predictive toxicology. | |
Record name | (3R,6R)-3,6-dimethyl-1,4-dioxane-2,5-dione | |
Source | European Chemicals Agency (ECHA) | |
URL | https://echa.europa.eu/substance-information/-/substanceinfo/100.115.631 | |
Description | The European Chemicals Agency (ECHA) is an agency of the European Union which is the driving force among regulatory authorities in implementing the EU's groundbreaking chemicals legislation for the benefit of human health and the environment as well as for innovation and competitiveness. | |
Explanation | Use of the information, documents and data from the ECHA website is subject to the terms and conditions of this Legal Notice, and subject to other binding limitations provided for under applicable law, the information, documents and data made available on the ECHA website may be reproduced, distributed and/or used, totally or in part, for non-commercial purposes provided that ECHA is acknowledged as the source: "Source: European Chemicals Agency, http://echa.europa.eu/". Such acknowledgement must be included in each copy of the material. ECHA permits and encourages organisations and individuals to create links to the ECHA website under the following cumulative conditions: Links can only be made to webpages that provide a link to the Legal Notice page. | |
Record name | LACTIDE, D- | |
Source | FDA Global Substance Registration System (GSRS) | |
URL | https://gsrs.ncats.nih.gov/ginas/app/beta/substances/I1863O7V0Q | |
Description | The FDA Global Substance Registration System (GSRS) enables the efficient and accurate exchange of information on what substances are in regulated products. Instead of relying on names, which vary across regulatory domains, countries, and regions, the GSRS knowledge base makes it possible for substances to be defined by standardized, scientific descriptions. | |
Explanation | Unless otherwise noted, the contents of the FDA website (www.fda.gov), both text and graphics, are not copyrighted. They are in the public domain and may be republished, reprinted and otherwise used freely by anyone without the need to obtain permission from FDA. Credit to the U.S. Food and Drug Administration as the source is appreciated but not required. | |
Synthesis routes and methods I
Procedure details
Synthesis routes and methods II
Procedure details
Retrosynthesis Analysis
AI-Powered Synthesis Planning: Our tool employs the Template_relevance Pistachio, Template_relevance Bkms_metabolic, Template_relevance Pistachio_ringbreaker, Template_relevance Reaxys, Template_relevance Reaxys_biocatalysis model, leveraging a vast database of chemical reactions to predict feasible synthetic routes.
One-Step Synthesis Focus: Specifically designed for one-step synthesis, it provides concise and direct routes for your target compounds, streamlining the synthesis process.
Accurate Predictions: Utilizing the extensive PISTACHIO, BKMS_METABOLIC, PISTACHIO_RINGBREAKER, REAXYS, REAXYS_BIOCATALYSIS database, our tool offers high-accuracy predictions, reflecting the latest in chemical research and data.
Strategy Settings
Precursor scoring | Relevance Heuristic |
---|---|
Min. plausibility | 0.01 |
Model | Template_relevance |
Template Set | Pistachio/Bkms_metabolic/Pistachio_ringbreaker/Reaxys/Reaxys_biocatalysis |
Top-N result to add to graph | 6 |
Feasible Synthetic Routes
Q1: What are the key thermal properties of polylactides synthesized from D-lactide?
A1: Poly(D-lactide) (PDLA) exhibits similar thermal properties to its enantiomer, poly(L-lactide) (PLLA). Both have a glass transition temperature (Tg) that can be influenced by factors like molecular weight and D-lactide content. [] Importantly, PDLA can form stereocomplexes with PLLA, resulting in significantly enhanced thermal stability, with melting temperatures (Tm) around 50 °C higher than either homopolymer. []
Q2: How does the D-lactide content affect the properties of polylactide materials?
A2: Increasing the D-lactide content generally decreases the crystallinity of PLA. High D-lactide content can lead to amorphous PLA, while lower contents allow for semi-crystalline structures. [, , ] This directly impacts properties like flexibility, degradation rate, and mechanical strength. For instance, higher D-lactide content can enhance flexibility but may reduce strength. [, ]
Q3: Can D-lactide based polymers be used for biomedical applications?
A3: Yes, poly(D-lactide) and its copolymers are promising for biomedical applications due to their biocompatibility and biodegradability. [, ] For example, poly(D-lactide) membranes have shown potential in bone regeneration by promoting bone formation within defects. []
Q4: What is the impact of stereocomplex formation on the properties of PLA materials?
A4: Stereocomplex formation between PDLA and PLLA leads to enhanced thermal and mechanical properties compared to the individual homopolymers. [, , , , ] This is due to the formation of a more tightly packed and thermodynamically stable crystalline structure.
Q5: How does the molecular weight of PDLA influence the properties of PLA blends?
A5: Blending PLLA with supramolecular polymers based on PDLA-PCVL-PDLA triblock copolymers, where PCVL represents poly(ε-caprolactone-co-δ-valerolactone), has been shown to improve PLLA properties. [] The molecular weight of the PDLA block in the supramolecular polymer influences the crystallization rate, melt strength, and toughness of the blend.
Q6: What makes electrospun nanofiber mats from D-lactide-containing PLAs unique?
A6: Electrospinning PLAs with varying D-lactide contents offers control over nanofiber morphology and mat properties. [] Higher D-lactide content can lead to coarser fibers with higher water vapor transmission rates, making them suitable for specific applications like wound dressings.
Q7: What are the common methods for synthesizing poly(D-lactide)?
A7: PDLA is typically synthesized via ring-opening polymerization (ROP) of D-lactide, often using catalysts like tin(II) octoate (Sn(Oct)2). [, , , ] This polymerization can be achieved in bulk or solution, with reaction conditions influencing the final polymer properties.
Q8: Can enzymes be used to catalyze the polymerization of D-lactide?
A8: Yes, engineered enzymes like Candida antarctica lipase B have been successfully redesigned to catalyze the ring-opening polymerization of D-lactide, offering a greener alternative to traditional catalysts. []
Q9: How can the molecular weight of PDLA be controlled during synthesis?
A9: The molecular weight of PDLA can be controlled by factors like monomer-to-initiator ratio, reaction time, and temperature. [, ] Chain extenders can also be added during polymerization to increase molecular weight. []
Q10: How is the stereocomplex formation between PLLA and PDLA confirmed?
A10: Stereocomplex formation is confirmed by various techniques, including differential scanning calorimetry (DSC), which shows a higher melting temperature for the stereocomplex compared to individual polymers. [, , , , ] Other techniques include wide-angle X-ray diffraction (WAXD), which reveals a distinct crystalline structure for the stereocomplex, and Fourier-transform infrared spectroscopy (FTIR). [, , , ]
Q11: How can we visualize the aggregates formed by stereocomplexation?
A11: Advanced microscopy techniques, like confocal laser scanning fluorescence microscopy (CLSFM), are used to visualize the aggregates. This is often done by using fluorescently labeled PDLA, allowing researchers to study the size, shape, and distribution of the aggregates. [, ]
Q12: What are the spectral characteristics of D-lactide?
A12: D-lactide can be characterized using spectroscopic techniques like FTIR and NMR. FTIR helps identify functional groups, while 1H-NMR and 13C-NMR provide detailed information about the arrangement of atoms within the molecule. [, ]
Q13: What are the limitations of conventional linear high-molecular-weight stereocomplex PLA?
A13: One limitation is its limited melt stability, meaning it may not fully regain its stereocomplex structure after melting, affecting its processability. []
Q14: Are there strategies to overcome the melt stability issue of stereocomplex PLA?
A14: Yes, incorporating small amounts of ε-caprolactone into PDLA, forming a random copolymer (PDLCL), has shown promise in enhancing the melt stability of stereocomplex PLA. [] The ε-caprolactone acts as a soft segment, facilitating chain movement and promoting stereocomplex re-formation after melting.
Q15: What is the significance of controlling the degradation rate of D-lactide-based materials?
A15: Controlling degradation is crucial for biomedical applications. The degradation rate can be tailored by adjusting factors like molecular weight, crystallinity, and copolymer composition. [, ]
Q16: How can the mechanical properties of PLA be further enhanced?
A16: Incorporating reinforcing agents like carbon nanotubes or nanoclays into the PLA matrix can significantly enhance mechanical properties, including tensile strength and toughness. [, ] Surface modification of these reinforcing agents can further improve their dispersion and interfacial adhesion within the PLA matrix.
Disclaimer and Information on In-Vitro Research Products
Please be aware that all articles and product information presented on BenchChem are intended solely for informational purposes. The products available for purchase on BenchChem are specifically designed for in-vitro studies, which are conducted outside of living organisms. In-vitro studies, derived from the Latin term "in glass," involve experiments performed in controlled laboratory settings using cells or tissues. It is important to note that these products are not categorized as medicines or drugs, and they have not received approval from the FDA for the prevention, treatment, or cure of any medical condition, ailment, or disease. We must emphasize that any form of bodily introduction of these products into humans or animals is strictly prohibited by law. It is essential to adhere to these guidelines to ensure compliance with legal and ethical standards in research and experimentation.