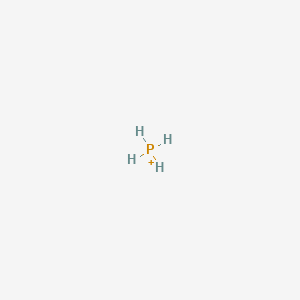
Phosphonium
Overview
Description
Phosphorane is a phosphorus hydride consisting of a single pentavalent phosphorus carrying five hydrogens. The parent hydride of the phosphorane class. It is a member of phosphoranes, a phosphorus hydride and a mononuclear parent hydride.
Scientific Research Applications
Transport Properties and Ionicity
Phosphonium ionic liquids (ILs) are promising electrolytes in both basic and applied research. Studies by Philippi et al. (2017) synthesized novel this compound ILs, investigating their important transport properties such as viscosity, conductivity, and diffusivity. This research contributes to a better understanding of IL properties based on molecular composition, facilitating the optimization of ILs for practical applications.
Mitochondrial Bioenergetics and Free Radical Biology
Lipophilic this compound cations have been significant tools for exploring mitochondrial bioenergetics and free radical biology. As outlined by Ross et al. (2005), these molecules have been extensively used since the late 1960s to study mitochondrial functions.
Removal of Metal Ions
This compound ionic liquids are increasingly used for metal ion separation. Research by Regel-Rosocka et al. (2012) demonstrated the successful application of two quaternary this compound salts in removing Zn(II) and iron ions from chloride solutions, showing their effectiveness as extractants.
Polyelectrolytes and Ionic Liquids
This compound cation-based ionic liquids often offer superior properties compared to ammonium cation-based ILs, with applications in batteries, super-capacitors, and corrosion protection. Jangu and Long (2014) discuss how polymerized ionic liquids and polyelectrolytes play major roles in biological applications such as antimicrobials and drug delivery.
Environmental Chemistry
Phosphonates, closely related to this compound, are used as chelating agents and scale inhibitors. The study by Nowack (2003) emphasizes the unique properties of phosphonates that affect their environmental behavior, highlighting their strong interaction with surfaces and significant removal in technical and natural systems.
Antimicrobial and Anti-Electrostatic Properties
This compound ionic liquids have been tested for antimicrobial activity and anti-electrostatic properties. Cieniecka-Rosłonkiewicz et al. (2005) found that both the cation structure and the type of anion in these ionic liquids affect their biological activity.
Ionic Liquids Overview
An overview of this compound-based ionic liquids and their properties is provided by Fraser and Macfarlane (2009). They discuss the applications of these ILs in extraction solvents, chemical synthesis solvents, and in corrosion protection, among others.
Gene Delivery
This compound salt-containing polymers have emerged as attractive materials for non-viral gene delivery systems. Rose, Mastrotto, and Mantovani (2017) highlight how these materials can enhance the binding of nucleic acids at lower concentrations, mediate good transfection efficiency, and exhibit low cytotoxicity.
Properties
CAS No. |
16749-13-6 |
---|---|
Molecular Formula |
H4P+ |
Molecular Weight |
35.006 g/mol |
IUPAC Name |
phosphanium |
InChI |
InChI=1S/H3P/h1H3/p+1 |
InChI Key |
XYFCBTPGUUZFHI-UHFFFAOYSA-O |
SMILES |
[PH4+] |
Canonical SMILES |
[PH4+] |
16749-13-6 | |
Synonyms |
Phosphine conjugate acid |
Origin of Product |
United States |
Synthesis routes and methods I
Procedure details
Synthesis routes and methods II
Procedure details
Synthesis routes and methods III
Procedure details
Disclaimer and Information on In-Vitro Research Products
Please be aware that all articles and product information presented on BenchChem are intended solely for informational purposes. The products available for purchase on BenchChem are specifically designed for in-vitro studies, which are conducted outside of living organisms. In-vitro studies, derived from the Latin term "in glass," involve experiments performed in controlled laboratory settings using cells or tissues. It is important to note that these products are not categorized as medicines or drugs, and they have not received approval from the FDA for the prevention, treatment, or cure of any medical condition, ailment, or disease. We must emphasize that any form of bodily introduction of these products into humans or animals is strictly prohibited by law. It is essential to adhere to these guidelines to ensure compliance with legal and ethical standards in research and experimentation.