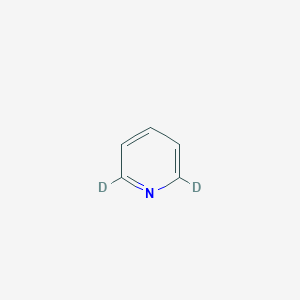
Pyridine-2,6-d2
Overview
Description
Pyridine-2,6-d2 is a deuterated derivative of pyridine, where two hydrogen atoms at the 2 and 6 positions of the pyridine ring are replaced by deuterium atoms. This compound is of significant interest in various fields of scientific research due to its unique isotopic properties, which can be utilized in spectroscopic studies and kinetic isotope effect investigations.
Mechanism of Action
Target of Action
It has been used in the synthesis of new heterocyclic polyurethane materials . It’s also been reported to have significant roles in coordination chemistry, stabilization of reactive species, synthetic modeling of some metalloenzyme active sites, catalytic organic transformations, and sensing .
Mode of Action
It has been reported that the inclusion of the pyridine derivative into polyurethane urea chain structure had been successful . The obtained polyurethane matrix structure was characterized through fewer specific intermolecular hydrogen bonds between the pyridine units, urethane, and amide groups .
Biochemical Pathways
It has been involved in the synthesis of new heterocyclic polyurethane materials . It’s also been reported to have roles in coordination chemistry, stabilization of reactive species, synthetic modeling of some metalloenzyme active sites, catalytic organic transformations, and sensing .
Pharmacokinetics
A study reported that a polymer-interferon alpha conjugate synthesized using pyridine-2,6-dicarboxaldehyde showed significantly improved pharmacokinetics .
Result of Action
It has been reported that the introduction of 2,6-pyridinedicarboxamide into the main chains of linear and crosslinked polyurethanes resulted in materials with improved thermal stability compared with conventional polyurethanes .
Action Environment
A study reported that a light-conversion agent synthesized using pyridine-2,6-dicarboxaldehyde showed high compatibility and stability for light-conversion agricultural films .
Preparation Methods
Synthetic Routes and Reaction Conditions: The synthesis of Pyridine-2,6-d2 typically involves the deuteration of pyridine. One common method is the exchange of hydrogen atoms with deuterium using deuterium oxide (D2O) in the presence of a catalyst. The reaction is usually carried out under reflux conditions to ensure complete exchange of hydrogen atoms at the desired positions.
Industrial Production Methods: Industrial production of this compound follows similar principles but on a larger scale. The process involves the use of high-purity deuterium oxide and optimized reaction conditions to achieve high yields and purity. The use of continuous flow reactors and advanced catalytic systems can enhance the efficiency and scalability of the production process.
Chemical Reactions Analysis
Types of Reactions: Pyridine-2,6-d2 undergoes various types of chemical reactions, including:
Oxidation: this compound can be oxidized to form pyridine N-oxide derivatives.
Reduction: Reduction reactions can convert this compound to piperidine derivatives.
Substitution: Electrophilic and nucleophilic substitution reactions can introduce various functional groups at different positions on the pyridine ring.
Common Reagents and Conditions:
Oxidation: Common oxidizing agents include hydrogen peroxide and peracids.
Reduction: Reducing agents such as lithium aluminum hydride or catalytic hydrogenation are used.
Substitution: Reagents like halogens, alkyl halides, and organometallic compounds are commonly employed.
Major Products:
Oxidation: Pyridine N-oxide derivatives.
Reduction: Piperidine derivatives.
Substitution: Various substituted pyridine derivatives depending on the reagents used.
Scientific Research Applications
Pyridine-2,6-d2 has a wide range of applications in scientific research:
Chemistry: Used as a solvent and reagent in organic synthesis. Its deuterated form is valuable in nuclear magnetic resonance (NMR) spectroscopy for studying reaction mechanisms and molecular structures.
Biology: Employed in isotope labeling studies to trace metabolic pathways and enzyme mechanisms.
Medicine: Utilized in the development of deuterated drugs, which can exhibit improved pharmacokinetic properties.
Industry: Applied in the production of specialty chemicals and materials with enhanced properties due to the presence of deuterium.
Comparison with Similar Compounds
Pyridine: The non-deuterated parent compound.
Pyridine-2,6-dicarboxamide: A derivative with carboxamide groups at the 2 and 6 positions.
Pyridine-2,6-dicarboxylic acid: A derivative with carboxylic acid groups at the 2 and 6 positions.
Comparison: Pyridine-2,6-d2 is unique due to the presence of deuterium atoms, which impart distinct isotopic properties. This makes it particularly valuable in spectroscopic studies and kinetic isotope effect investigations, where the behavior of deuterium can provide additional insights compared to its non-deuterated counterparts. The other similar compounds, while structurally related, do not offer the same isotopic advantages and are used for different applications based on their functional groups.
Properties
IUPAC Name |
2,6-dideuteriopyridine | |
---|---|---|
Source | PubChem | |
URL | https://pubchem.ncbi.nlm.nih.gov | |
Description | Data deposited in or computed by PubChem | |
InChI |
InChI=1S/C5H5N/c1-2-4-6-5-3-1/h1-5H/i4D,5D | |
Source | PubChem | |
URL | https://pubchem.ncbi.nlm.nih.gov | |
Description | Data deposited in or computed by PubChem | |
InChI Key |
JUJWROOIHBZHMG-KFRNQKGQSA-N | |
Source | PubChem | |
URL | https://pubchem.ncbi.nlm.nih.gov | |
Description | Data deposited in or computed by PubChem | |
Canonical SMILES |
C1=CC=NC=C1 | |
Source | PubChem | |
URL | https://pubchem.ncbi.nlm.nih.gov | |
Description | Data deposited in or computed by PubChem | |
Isomeric SMILES |
[2H]C1=NC(=CC=C1)[2H] | |
Source | PubChem | |
URL | https://pubchem.ncbi.nlm.nih.gov | |
Description | Data deposited in or computed by PubChem | |
Molecular Formula |
C5H5N | |
Source | PubChem | |
URL | https://pubchem.ncbi.nlm.nih.gov | |
Description | Data deposited in or computed by PubChem | |
Molecular Weight |
81.11 g/mol | |
Source | PubChem | |
URL | https://pubchem.ncbi.nlm.nih.gov | |
Description | Data deposited in or computed by PubChem | |
Synthesis routes and methods I
Procedure details
Synthesis routes and methods II
Procedure details
Synthesis routes and methods III
Procedure details
Synthesis routes and methods IV
Procedure details
Synthesis routes and methods V
Procedure details
Retrosynthesis Analysis
AI-Powered Synthesis Planning: Our tool employs the Template_relevance Pistachio, Template_relevance Bkms_metabolic, Template_relevance Pistachio_ringbreaker, Template_relevance Reaxys, Template_relevance Reaxys_biocatalysis model, leveraging a vast database of chemical reactions to predict feasible synthetic routes.
One-Step Synthesis Focus: Specifically designed for one-step synthesis, it provides concise and direct routes for your target compounds, streamlining the synthesis process.
Accurate Predictions: Utilizing the extensive PISTACHIO, BKMS_METABOLIC, PISTACHIO_RINGBREAKER, REAXYS, REAXYS_BIOCATALYSIS database, our tool offers high-accuracy predictions, reflecting the latest in chemical research and data.
Strategy Settings
Precursor scoring | Relevance Heuristic |
---|---|
Min. plausibility | 0.01 |
Model | Template_relevance |
Template Set | Pistachio/Bkms_metabolic/Pistachio_ringbreaker/Reaxys/Reaxys_biocatalysis |
Top-N result to add to graph | 6 |
Feasible Synthetic Routes
Q1: Why is the study of pyridine-2,6-d2 important in vibrational spectroscopy?
A: Isotopic substitution, like replacing hydrogen with deuterium in this compound, causes predictable shifts in vibrational frequencies. These shifts help researchers assign vibrational modes to specific atomic motions within the molecule. By comparing the spectra of pyridine and its deuterated analogs, a more complete understanding of pyridine's vibrational behavior emerges. [, , ]
Q2: What are some of the key spectroscopic techniques used to study this compound?
A: Researchers utilize a combination of infrared (IR) and Raman spectroscopy to analyze the vibrational characteristics of this compound. IR spectroscopy reveals information about changes in dipole moment during molecular vibrations, while Raman spectroscopy probes polarizability changes. The combined data from these techniques, including depolarization ratios obtained from Raman measurements, offer a comprehensive picture of molecular vibrations. [, , ]
Q3: How does the vibrational assignment of this compound compare to that of pyridine?
A: While the overall vibrational patterns share similarities, the isotopic substitution in this compound necessitates adjustments to the vibrational assignments compared to pyridine. These adjustments primarily involve the B2 symmetry modes and are crucial for achieving consistency with observed spectroscopic data and theoretical calculations. [, ]
Q4: How has computational chemistry contributed to our understanding of this compound's vibrational behavior?
A: Computational models, employing methods like normal coordinate analysis with modified Urey-Bradley force fields, have been instrumental in refining the vibrational assignments of this compound. [] These calculations provide insights into the force constants associated with specific bonds and angles within the molecule, allowing for a more accurate prediction and interpretation of vibrational frequencies.
Q5: Beyond fundamental vibrational analysis, how else has this compound been utilized in research?
A: this compound has proven valuable in surface-enhanced Raman scattering (SERS) studies. Researchers observed SERS spectra of this compound in silver borohydride sols, providing insights into adsorption mechanisms and the role of aggregation in SERS enhancement. [] This research highlights the potential of this compound as a probe molecule in surface science.
Disclaimer and Information on In-Vitro Research Products
Please be aware that all articles and product information presented on BenchChem are intended solely for informational purposes. The products available for purchase on BenchChem are specifically designed for in-vitro studies, which are conducted outside of living organisms. In-vitro studies, derived from the Latin term "in glass," involve experiments performed in controlled laboratory settings using cells or tissues. It is important to note that these products are not categorized as medicines or drugs, and they have not received approval from the FDA for the prevention, treatment, or cure of any medical condition, ailment, or disease. We must emphasize that any form of bodily introduction of these products into humans or animals is strictly prohibited by law. It is essential to adhere to these guidelines to ensure compliance with legal and ethical standards in research and experimentation.