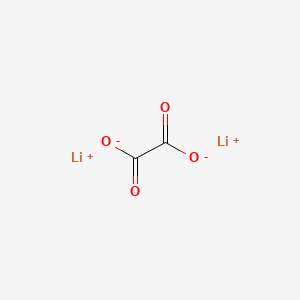
草酸锂
描述
Synthesis Analysis
The synthesis of lithium oxalate involves various methodologies, aiming at optimizing its physical and chemical properties for specific applications. For instance, the synthesis of iron oxalate nanoparticles has been explored as anode materials for lithium-ion batteries (LIBs), demonstrating the influence of synthesis conditions on the crystal structure and performance of the resulting materials (Zhang et al., 2020). Similarly, the synthesis of lithium difluoro(oxalato)borate (LiDFOB) provides insights into the structural characterization and properties of lithium oxalate derivatives, highlighting the critical role of synthesis routes in determining the crystal structure and electrochemical properties (Allen et al., 2011).
Molecular Structure Analysis
The molecular structure of lithium oxalate and its derivatives significantly influences their chemical reactivity and application potential. The crystal structure of lithium difluoro(oxalato)borate, for example, reveals a highly symmetric orthorhombic structure, providing a foundation for understanding the interactions within lithium battery electrolytes (Allen et al., 2011). Such structural insights are crucial for tailoring lithium oxalate compounds for specific applications, including energy storage and conversion.
Chemical Reactions and Properties
Lithium oxalate participates in various chemical reactions, contributing to its diverse applications. The reactivity of lithium oxalate and its derivatives, such as in the formation of iron oxalate as anode materials for LIBs, underscores the importance of chemical properties in determining the suitability of these compounds for energy storage applications (Zhang et al., 2020). Understanding these chemical reactions and properties is essential for the development of advanced materials for lithium-ion batteries and other technologies.
Physical Properties Analysis
The physical properties of lithium oxalate, such as morphology, crystallinity, and thermal stability, are key factors influencing its application in various fields. Research on iron oxalate for lithium-ion batteries highlights the impact of physical properties on the electrochemical performance, demonstrating the relationship between morphology, phase structure, and battery capacity (Zhang et al., 2020). Such analyses are crucial for optimizing lithium oxalate-based materials for specific technological applications.
Chemical Properties Analysis
The chemical properties of lithium oxalate, including reactivity, solubility, and electrochemical behavior, play a significant role in its utilization in various applications. The study of lithium difluoro(oxalato)borate and its impact on the electrochemical performances of lithium-ion batteries illustrates the importance of chemical properties in enhancing battery performance and stability (Allen et al., 2011). Such insights into chemical properties are essential for the development of high-performance materials for energy storage and other applications.
科学研究应用
锂离子电池
草酸锂是一种很有吸引力的锂离子电池 (LIB) 正极预锂化添加剂。然而,其高分解电位(>4.7 V)阻碍了其应用。 由于 NaNO2 添加剂和 LiNi0.83Co0.07Mn0.1O2 (NCM) 正极材料的液固协同效应,微米级 Li2C2O4 在 4.3 V 的低充电截止电压下分解效率达到 100% .
电解质添加剂
双草酸硼酸锂 (LiBOB) 是 LIB 中最常用的成膜电解质添加剂之一。 它可以形成致密的含硼聚合物作为固体电解质中间层(或正极电解质中间层),以隔离电极材料和电解质,防止副反应 .
金属沉淀
草酸锂可用于从锂离子电池浸出液中选择性沉淀金属草酸盐。 此过程对于从废旧锂离子电池中回收钴和镍等贱金属至关重要 .
作用机制
Target of Action
Lithium Oxalate (Li2C2O4) is a salt of lithium metal and oxalic acid . It consists of lithium cations (Li+) and oxalate anions (C2O4^2-) . The primary targets of Lithium Oxalate are the biochemical pathways where these ions play a significant role.
Mode of Action
Lithium Oxalate interacts with its targets primarily through ionic interactions. The lithium cations (Li+) and oxalate anions (C2O4^2-) derived from Lithium Oxalate can participate in various biochemical reactions. For instance, in lithium-ion batteries, Lithium Oxalate has shown potential as a lithium-compensation material due to its high theoretical capacity, low cost, and air stability .
Biochemical Pathways
Lithium has been known to affect several signaling pathways that overlap with those regulated by mglur . For example, group 1 mGluRs mediate cerebellar mGluR-LTD by increasing inositol triphosphate (IP3) generation and releasing intracellular Ca2+ stores, and lithium is able to reverse these effects by inhibiting inositol levels .
Pharmacokinetics
Lithium Oxalate is soluble in water , which suggests that it could be readily absorbed and distributed in the body It’s important to note that the pharmacokinetic properties can significantly impact the bioavailability and overall effectiveness of the compound.
Result of Action
The molecular and cellular effects of Lithium Oxalate’s action are largely dependent on the specific context and the biochemical pathways it interacts with. In the context of lithium-ion batteries, for example, Lithium Oxalate can enhance capacity retention and coulombic efficiency .
Action Environment
Environmental factors can significantly influence the action, efficacy, and stability of Lithium Oxalate. For instance, high relative humidity can accelerate the rate of increase in weight of lithium bis(oxalato)borate, a compound related to Lithium Oxalate . This suggests that environmental conditions such as humidity could potentially influence the stability and efficacy of Lithium Oxalate.
安全和危害
未来方向
属性
IUPAC Name |
dilithium;oxalate | |
---|---|---|
Source | PubChem | |
URL | https://pubchem.ncbi.nlm.nih.gov | |
Description | Data deposited in or computed by PubChem | |
InChI |
InChI=1S/C2H2O4.2Li/c3-1(4)2(5)6;;/h(H,3,4)(H,5,6);;/q;2*+1/p-2 | |
Source | PubChem | |
URL | https://pubchem.ncbi.nlm.nih.gov | |
Description | Data deposited in or computed by PubChem | |
InChI Key |
YNQRWVCLAIUHHI-UHFFFAOYSA-L | |
Source | PubChem | |
URL | https://pubchem.ncbi.nlm.nih.gov | |
Description | Data deposited in or computed by PubChem | |
Canonical SMILES |
[Li+].[Li+].C(=O)(C(=O)[O-])[O-] | |
Source | PubChem | |
URL | https://pubchem.ncbi.nlm.nih.gov | |
Description | Data deposited in or computed by PubChem | |
Molecular Formula |
C2Li2O4 | |
Source | PubChem | |
URL | https://pubchem.ncbi.nlm.nih.gov | |
Description | Data deposited in or computed by PubChem | |
DSSTOX Substance ID |
DTXSID4060288 | |
Record name | Lithium oxalate | |
Source | EPA DSSTox | |
URL | https://comptox.epa.gov/dashboard/DTXSID4060288 | |
Description | DSSTox provides a high quality public chemistry resource for supporting improved predictive toxicology. | |
Molecular Weight |
102.0 g/mol | |
Source | PubChem | |
URL | https://pubchem.ncbi.nlm.nih.gov | |
Description | Data deposited in or computed by PubChem | |
CAS RN |
553-91-3, 30903-87-8 | |
Record name | Lithium oxalate | |
Source | ChemIDplus | |
URL | https://pubchem.ncbi.nlm.nih.gov/substance/?source=chemidplus&sourceid=0000553913 | |
Description | ChemIDplus is a free, web search system that provides access to the structure and nomenclature authority files used for the identification of chemical substances cited in National Library of Medicine (NLM) databases, including the TOXNET system. | |
Record name | Ethanedioic acid, lithium salt (1:2) | |
Source | EPA Chemicals under the TSCA | |
URL | https://www.epa.gov/chemicals-under-tsca | |
Description | EPA Chemicals under the Toxic Substances Control Act (TSCA) collection contains information on chemicals and their regulations under TSCA, including non-confidential content from the TSCA Chemical Substance Inventory and Chemical Data Reporting. | |
Record name | Lithium oxalate | |
Source | EPA DSSTox | |
URL | https://comptox.epa.gov/dashboard/DTXSID4060288 | |
Description | DSSTox provides a high quality public chemistry resource for supporting improved predictive toxicology. | |
Record name | Dilithium oxalate | |
Source | European Chemicals Agency (ECHA) | |
URL | https://echa.europa.eu/substance-information/-/substanceinfo/100.008.232 | |
Description | The European Chemicals Agency (ECHA) is an agency of the European Union which is the driving force among regulatory authorities in implementing the EU's groundbreaking chemicals legislation for the benefit of human health and the environment as well as for innovation and competitiveness. | |
Explanation | Use of the information, documents and data from the ECHA website is subject to the terms and conditions of this Legal Notice, and subject to other binding limitations provided for under applicable law, the information, documents and data made available on the ECHA website may be reproduced, distributed and/or used, totally or in part, for non-commercial purposes provided that ECHA is acknowledged as the source: "Source: European Chemicals Agency, http://echa.europa.eu/". Such acknowledgement must be included in each copy of the material. ECHA permits and encourages organisations and individuals to create links to the ECHA website under the following cumulative conditions: Links can only be made to webpages that provide a link to the Legal Notice page. | |
Record name | Ethanedioic acid, lithium salt (1:?) | |
Source | European Chemicals Agency (ECHA) | |
URL | https://echa.europa.eu/substance-information/-/substanceinfo/100.107.577 | |
Description | The European Chemicals Agency (ECHA) is an agency of the European Union which is the driving force among regulatory authorities in implementing the EU's groundbreaking chemicals legislation for the benefit of human health and the environment as well as for innovation and competitiveness. | |
Explanation | Use of the information, documents and data from the ECHA website is subject to the terms and conditions of this Legal Notice, and subject to other binding limitations provided for under applicable law, the information, documents and data made available on the ECHA website may be reproduced, distributed and/or used, totally or in part, for non-commercial purposes provided that ECHA is acknowledged as the source: "Source: European Chemicals Agency, http://echa.europa.eu/". Such acknowledgement must be included in each copy of the material. ECHA permits and encourages organisations and individuals to create links to the ECHA website under the following cumulative conditions: Links can only be made to webpages that provide a link to the Legal Notice page. | |
Record name | LITHIUM OXALATE | |
Source | FDA Global Substance Registration System (GSRS) | |
URL | https://gsrs.ncats.nih.gov/ginas/app/beta/substances/K737OT0E73 | |
Description | The FDA Global Substance Registration System (GSRS) enables the efficient and accurate exchange of information on what substances are in regulated products. Instead of relying on names, which vary across regulatory domains, countries, and regions, the GSRS knowledge base makes it possible for substances to be defined by standardized, scientific descriptions. | |
Explanation | Unless otherwise noted, the contents of the FDA website (www.fda.gov), both text and graphics, are not copyrighted. They are in the public domain and may be republished, reprinted and otherwise used freely by anyone without the need to obtain permission from FDA. Credit to the U.S. Food and Drug Administration as the source is appreciated but not required. | |
Retrosynthesis Analysis
AI-Powered Synthesis Planning: Our tool employs the Template_relevance Pistachio, Template_relevance Bkms_metabolic, Template_relevance Pistachio_ringbreaker, Template_relevance Reaxys, Template_relevance Reaxys_biocatalysis model, leveraging a vast database of chemical reactions to predict feasible synthetic routes.
One-Step Synthesis Focus: Specifically designed for one-step synthesis, it provides concise and direct routes for your target compounds, streamlining the synthesis process.
Accurate Predictions: Utilizing the extensive PISTACHIO, BKMS_METABOLIC, PISTACHIO_RINGBREAKER, REAXYS, REAXYS_BIOCATALYSIS database, our tool offers high-accuracy predictions, reflecting the latest in chemical research and data.
Strategy Settings
Precursor scoring | Relevance Heuristic |
---|---|
Min. plausibility | 0.01 |
Model | Template_relevance |
Template Set | Pistachio/Bkms_metabolic/Pistachio_ringbreaker/Reaxys/Reaxys_biocatalysis |
Top-N result to add to graph | 6 |
Feasible Synthetic Routes
Q & A
Q1: What is the role of lithium oxalate in lithium-ion batteries?
A1: Lithium oxalate serves as a sacrificial salt and electrolyte additive in lithium-ion batteries [, , , ]. Its decomposition during the first charging cycle contributes to the formation of a stable solid electrolyte interphase (SEI) on the anode surface [, , ]. This SEI layer is crucial for battery performance, as it facilitates lithium-ion transport while preventing further electrolyte decomposition and enhancing cycling stability [].
Q2: How does lithium oxalate improve the lifespan of anode-free lithium metal batteries (AFLMBs)?
A2: Lithium oxalate acts as a lithium reservoir when added to the cathode of AFLMBs []. This reservoir helps mitigate the issue of dendrite and dead-lithium buildup, which typically plague AFLMBs and lead to short lifespans. Cells incorporating lithium oxalate demonstrated significantly improved capacity retention compared to those without [].
Q3: Can lithium oxalate improve the performance of high-voltage lithium-ion batteries?
A3: Research suggests that lithium oxalate, when used as an additive in methyl butyrate-based electrolytes, can improve the rate capability of Li-ion cells, even at low temperatures [, ]. This enhancement is particularly beneficial for high-voltage systems utilizing Li(1.2)Ni(0.15)Co(0.10)Mn(0.55)O2 cathodes [].
Q4: Does lithium oxalate exhibit flame-retardant properties in lithium-ion batteries?
A4: Yes, studies have shown that incorporating lithium oxalate into graphite anodes can provide a flame-retardant effect []. This effect is attributed to the release of carbon dioxide (CO2) gas upon lithium oxalate decomposition [].
Q5: How does the presence of carbon dioxide, generated from lithium oxalate decomposition, impact silicon-graphite (SiG) anodes?
A5: Carbon dioxide, produced during the initial oxidation of lithium oxalate, acts as an effective SEI-forming additive for SiG anodes []. This additive contributes to better capacity retention and higher coulombic efficiency in lithium nickel manganese spinel (LNMO)/SiG full-cells, particularly when combined with fluoroethylene carbonate (FEC) in the electrolyte solution [].
Q6: What is the molecular formula and weight of lithium oxalate?
A6: The molecular formula of lithium oxalate is Li2C2O4, and its molecular weight is 101.90 g/mol.
Q7: How can spectroscopic techniques be used to study lithium oxalate and its derivatives?
A7: Several spectroscopic methods are employed to characterize lithium oxalate and its reactions. These include:
- Fourier Transform Infrared (FTIR) Spectroscopy: Identifies functional groups and analyzes the structure of lithium oxalate and its derivatives [, , ].
- Raman Spectroscopy: Provides complementary information to FTIR, especially for symmetrical vibrations and lattice modes in crystalline lithium oxalate [].
- Nuclear Magnetic Resonance (NMR) Spectroscopy: Characterizes the structure of lithium oxalate derivatives in solution [, ] and investigates lithium storage mechanisms in carbon materials [, ].
- X-ray Photoelectron Spectroscopy (XPS): Analyzes the elemental composition and chemical states of elements present in SEI layers containing lithium oxalate [, , ].
- Soft X-ray Spectroscopy: Provides detailed information about the electronic structure and bonding environment of elements in SEI layers containing lithium oxalate [].
Q8: Does lithium oxalate play a role in CO2 capture and conversion?
A8: Research has shown that a dinuclear copper(I) complex can electrocatalytically convert CO2 to oxalate with high selectivity, even in the presence of oxygen []. The oxalate can then be precipitated as lithium oxalate and the copper complex regenerated electrochemically, demonstrating a preliminary catalytic cycle for CO2 fixation [].
Q9: Can computational methods help understand the role of lithium oxalate in batteries?
A9: Yes, density functional theory (DFT) calculations are employed to investigate various aspects:
- Reaction Mechanisms: DFT helps elucidate the formation mechanism and stability of lithium oxalate as a discharge product in Li-CO2 batteries, revealing its potential in preventing the formation of lithium carbonate (Li2CO3) [].
- Catalytic Activity: DFT calculations can be used to evaluate the catalytic activities of various materials toward the discharge and charge processes in Li-CO2 batteries, identifying potential catalysts that promote lithium oxalate formation [].
- Solubility Predictions: Computer simulations, particularly molecular dynamics simulations, are used to predict the solubility of various lithium salts, including lithium oxalate, in different battery electrolyte solvents. These simulations can help understand SEI layer stability and battery capacity fade during storage [].
Q10: How does the solubility of lithium oxalate in organic solvents impact its applications?
A10: The solubility of lithium oxalate in organic solvents, such as dimethyl carbonate (DMC), is a critical factor affecting its behavior in lithium-ion batteries []. Studies have shown that lithium oxalate exhibits low solubility in DMC compared to other lithium salts found in SEI layers []. This low solubility, while potentially limiting its ability to migrate and form a robust SEI, can also be beneficial in preventing excessive SEI growth and maintaining long-term battery stability.
Q11: Can lithium oxalate be recovered and recycled from lithium-ion battery waste?
A11: Yes, lithium oxalate can be recovered from waste lithium-ion batteries through hydrometallurgical processes. For instance, oxalic acid leaching has been shown to be effective in extracting lithium oxalate from spent battery materials with high recovery rates []. This recovered lithium oxalate can then be potentially purified and reused in the production of new battery materials.
Q12: What are some essential resources for lithium oxalate research?
A12: Effective research on lithium oxalate requires access to various resources, including:
- Analytical Instruments: As discussed earlier, techniques like FTIR, Raman, NMR, XPS, and soft X-ray spectroscopy are essential for characterizing lithium oxalate and its reactions [, , , , , , ].
- Electrochemical Testing Equipment: Tools like potentiostats/galvanostats and battery cyclers are crucial for studying the electrochemical performance of lithium oxalate-containing battery systems [, , , , , ].
- Computational Resources: High-performance computing clusters and software packages for DFT calculations and molecular dynamics simulations are essential for theoretical studies of lithium oxalate in various applications [, ].
Q13: What are some examples of cross-disciplinary research involving lithium oxalate?
A13: Lithium oxalate research benefits from collaborations across disciplines, including:
- Materials Science & Engineering: Developing new battery materials and architectures that incorporate lithium oxalate effectively for improved performance and safety [, , , , , , , ].
- Chemistry: Synthesizing and characterizing novel lithium oxalate-based compounds with tailored properties for specific applications, such as electrolytes or additives [, ].
- Chemical Engineering: Optimizing lithium oxalate synthesis processes for scalability and cost-effectiveness, as well as developing efficient recycling and recovery methods from battery waste [, , , ].
- Environmental Science: Assessing the environmental impact of lithium oxalate production and disposal and developing sustainable manufacturing and recycling practices [].
体外研究产品的免责声明和信息
请注意,BenchChem 上展示的所有文章和产品信息仅供信息参考。 BenchChem 上可购买的产品专为体外研究设计,这些研究在生物体外进行。体外研究,源自拉丁语 "in glass",涉及在受控实验室环境中使用细胞或组织进行的实验。重要的是要注意,这些产品没有被归类为药物或药品,他们没有得到 FDA 的批准,用于预防、治疗或治愈任何医疗状况、疾病或疾病。我们必须强调,将这些产品以任何形式引入人类或动物的身体都是法律严格禁止的。遵守这些指南对确保研究和实验的法律和道德标准的符合性至关重要。