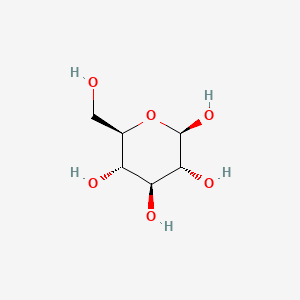
β-D-葡萄糖
描述
Synthesis Analysis
Curdlan synthesis involves several steps, starting from the regioselective modification of natural curdlan to introduce amino groups, a process critical for enhancing its functional properties. A significant method involves the regioselective bromination of curdlan, followed by nucleophilic substitution to introduce amino functionalities, which can further undergo O-acylation. This method demonstrates the potential for curdlan derivatives in biomedical applications (Zhang & Edgar, 2014). Additionally, the production of curdlan can be enhanced through specific fermentation strategies, such as nitrogen feeding, which has been shown to significantly increase curdlan yield in Rhizobium radiobacter (Wang et al., 2016).
Molecular Structure Analysis
The molecular structure of curdlan has been extensively studied, revealing its linear β-(1→3)-glucan nature. Techniques such as transcriptome profiling and membrane protein synthesis have provided insights into the biosynthesis mechanism and the enzymatic processes involved in curdlan polymerization. Specifically, the role of curdlan synthase (CrdS) and its integration into membranes for polymer synthesis highlights the complex biochemical pathways that govern curdlan production (Periasamy et al., 2013).
Chemical Reactions and Properties
Curdlan's ability to undergo various chemical reactions, such as esterification, phosphorylation, and the introduction of cationic groups, greatly expands its functional versatility. These modifications can significantly alter its physical and chemical properties, enabling the development of curdlan derivatives with tailored functionalities for specific applications. For instance, the synthesis of cationic 6-deoxy-6-(N,N,N-trialkylammonio)curdlan derivatives illustrates the potential for creating water-soluble curdlan derivatives suitable for biomedical applications (Zhang, Liu, & Edgar, 2016).
Physical Properties Analysis
The physical properties of curdlan, including its solubility, thermal stability, and gelation behavior, are significantly influenced by its molecular structure and the presence of any chemical modifications. Studies on curdlan derivatives, such as branched esters, have shown that the degree of substitution and the nature of the substituent groups can dramatically affect these properties, offering insights into the material properties of curdlan esters and their potential applications (Zhai, Danjo, & Iwata, 2018).
Chemical Properties Analysis
The chemical properties of curdlan, including its reactivity and interaction with other molecules, are crucial for its application in various fields. The synthesis of phosphorylated curdlan derivatives, for example, highlights the ability to introduce functional groups that can alter its polyelectrolyte behavior, enhancing its utility in diverse applications. The study of these derivatives provides valuable insights into the structure-property relationships of curdlan and its potential for functionalization (Suflet, Nicolescu, Popescu, & Chiţanu, 2011).
科学研究应用
抗糖尿病功能
β-D-葡萄糖,特别是β-葡聚糖,已被发现具有显著的抗糖尿病特性。 它们可以作为生物活性大分子,帮助控制 2 型糖尿病,并具有最小的副作用 {svg_1}.
酶生产
β-D-葡萄糖用于生产葡萄糖氧化酶,这种酶催化β-D-葡萄糖转化为葡萄糖酸和过氧化氢。 这种酶对于提高环境可持续性和粮食安全至关重要 {svg_2}.
生物燃料生产
β-葡萄糖苷酶,分解 β-D-葡萄糖的酶,已应用于木质纤维素生物质的糖化,用于生产生物乙醇 {svg_3}.
增强风味和营养质量
β-葡萄糖苷酶也用于葡萄酒和果汁生产,以增强风味 {svg_4}. 它们被添加到饲料中以改善农场动物对纤维素的消化 {svg_5}.
废纸回收
β-葡萄糖苷酶在废纸回收中得到应用,为环境可持续性做出贡献 {svg_6}.
食品工业应用
柯德兰,一种 β-D-葡萄糖形式,用作食品工业中的凝胶剂。它已获得美国 食品药品监督管理局 {svg_7} 的批准,作为食品添加剂。 它正在食品中的脂肪替代研究中进行评估,例如香肠、肉饼和其他肉制品 {svg_8}.
制药应用
柯德兰由于其独特的物理化学和流变特性,在制药行业有着广泛的应用 {svg_9}. 它已被进一步探索其潜在的治疗应用,在临床前环境中表现出免疫调节和抗肿瘤活性 {svg_10}.
治疗干预
柯德兰已显示出作为人类抗癌、抗疟疾、抗病毒和抗菌剂的潜力 {svg_11}. 它还表现出对人类免疫缺陷病毒 (HIV) 和埃及伊蚊病毒的有效抗病毒作用 {svg_12}.
作用机制
Target of Action
Beta-D-Glucose, also known as Beta-D-Glucopyranose or Curdlan, interacts with a variety of targets in the human body. These targets include DNA, Chondroitinase-B, Endoglucanase 5A, Lactase-like protein, Glycogen phosphorylase, Xylose isomerase, Glucokinase, Ferrichrome-iron receptor, and Hexokinase-1 . These targets play crucial roles in various biological processes, including energy metabolism, carbohydrate metabolism, and cellular signaling.
Mode of Action
Beta-D-Glucose primarily acts as an energy source for living organisms . It interacts with its targets, such as Glucokinase and Hexokinase-1, to initiate the process of glucose metabolism . This interaction results in the conversion of glucose into energy, which is then used by cells for various functions.
Biochemical Pathways
Beta-D-Glucose is involved in several biochemical pathways. It is the primary nutrient that controls insulin exocytosis . It is also involved in the glycolysis pathway, where it is converted into pyruvate, which may undergo further oxidation in the citric acid cycle . Additionally, it contributes to the polyol and hexosamine pathways .
Pharmacokinetics
It is known that beta-d-glucose is naturally occurring and is found in fruits and other parts of plants in its free state . It is used therapeutically in fluid and nutrient replacement .
Result of Action
The action of Beta-D-Glucose results in several molecular and cellular effects. It serves as a primary source of energy for living organisms, contributing to various metabolic processes . It also plays a role in insulin secretion, influencing the regulation of blood glucose levels . Furthermore, it is involved in the production of D-gluconic acid, which results in a decrease in pH, a decrease in oxygen availability, or the production of hydrogen peroxide .
Action Environment
Environmental factors can influence the action, efficacy, and stability of Beta-D-Glucose. For instance, environmental pollutants can disrupt beta cell function, potentially affecting the metabolism of Beta-D-Glucose . Lifestyle factors such as diet and physical activity can also impact the effectiveness of Beta-D-Glucose in managing blood glucose levels .
未来方向
Curdlan has exhibited immunoregulatory and antitumor activity in preclinical settings . It has been suggested that the virucidal properties of curdlans should be extended further for other deadly viruses, such as severe acute respiratory syndrome (SARS), Middle East respiratory syndrome (MERS), and the current severe acute respiratory syndrome coronavirus-2 (SARS-CoV-2/COVID-19) . The prebiotic property of curdlan would confer beneficial effects on the host by promoting the growth of healthy microbiota in the gut and consequently help to reduce gastrointestinal disorders . Therefore, curdlan can be employed in the manufacture of prebiotics for the management of various gastrointestinal dysbiosis problems .
生化分析
Biochemical Properties
Curdlan plays a crucial role in various biochemical reactions. It interacts with several enzymes, proteins, and other biomolecules. For instance, curdlan can be carboxymethylated to create a soluble polymer that serves as an excellent substrate for the assay of endo-1,3-β-D-glucanase . This interaction highlights curdlan’s role in enzymatic reactions, where it can be used to study the activity of specific enzymes. Additionally, curdlan’s interaction with proteins and other biomolecules can influence its gel strength and other physicochemical properties .
Cellular Effects
Curdlan has notable effects on various types of cells and cellular processes. It influences cell function by affecting cell signaling pathways, gene expression, and cellular metabolism. For example, curdlan’s role in biofilm formation and pathogen persistence is well-documented . It can also impact the immune response by evading the human immune system, thus playing a role in microbial survival and pathogenicity .
Molecular Mechanism
At the molecular level, curdlan exerts its effects through several mechanisms. It binds to specific biomolecules, leading to enzyme inhibition or activation and changes in gene expression. The stringent response signal, pppGpp, is indispensable for the transcriptional activation of curdlan biosynthesis . This highlights the complex regulatory mechanisms that control curdlan production and its subsequent effects on cellular processes.
Temporal Effects in Laboratory Settings
In laboratory settings, the effects of curdlan can change over time. Its stability and degradation are critical factors that influence its long-term effects on cellular function. Studies have shown that curdlan’s production is regulated by environmental cues, such as nitrogen starvation, which triggers the upregulation of the curdlan synthesis operon . This temporal regulation ensures that curdlan production is tightly controlled in response to changing environmental conditions.
Dosage Effects in Animal Models
The effects of curdlan vary with different dosages in animal models. At low doses, curdlan may have beneficial effects, such as enhancing immune responses or promoting wound healing. At high doses, curdlan can exhibit toxic or adverse effects, including inflammation or immune suppression . Understanding the dosage-dependent effects of curdlan is crucial for its safe and effective use in therapeutic applications.
Metabolic Pathways
Curdlan is involved in several metabolic pathways, interacting with various enzymes and cofactors. Its synthesis is regulated by transcriptional mechanisms that respond to environmental signals . Additionally, curdlan can influence metabolic flux and metabolite levels, further highlighting its role in cellular metabolism and biochemical reactions.
Transport and Distribution
Within cells and tissues, curdlan is transported and distributed through specific transporters and binding proteins. These interactions affect curdlan’s localization and accumulation, influencing its overall activity and function . Understanding the transport and distribution mechanisms of curdlan is essential for optimizing its use in various applications.
Subcellular Localization
Curdlan’s subcellular localization is directed by targeting signals and post-translational modifications. These mechanisms ensure that curdlan is directed to specific compartments or organelles, where it can exert its effects . The precise localization of curdlan within cells is critical for its function and effectiveness in biochemical and therapeutic applications.
属性
IUPAC Name |
6-(hydroxymethyl)oxane-2,3,4,5-tetrol | |
---|---|---|
Details | Computed by Lexichem TK 2.7.0 (PubChem release 2021.05.07) | |
Source | PubChem | |
URL | https://pubchem.ncbi.nlm.nih.gov | |
Description | Data deposited in or computed by PubChem | |
InChI |
InChI=1S/C6H12O6/c7-1-2-3(8)4(9)5(10)6(11)12-2/h2-11H,1H2 | |
Details | Computed by InChI 1.0.6 (PubChem release 2021.05.07) | |
Source | PubChem | |
URL | https://pubchem.ncbi.nlm.nih.gov | |
Description | Data deposited in or computed by PubChem | |
InChI Key |
WQZGKKKJIJFFOK-UHFFFAOYSA-N | |
Details | Computed by InChI 1.0.6 (PubChem release 2021.05.07) | |
Source | PubChem | |
URL | https://pubchem.ncbi.nlm.nih.gov | |
Description | Data deposited in or computed by PubChem | |
Canonical SMILES |
C(C1C(C(C(C(O1)O)O)O)O)O | |
Details | Computed by OEChem 2.3.0 (PubChem release 2021.05.07) | |
Source | PubChem | |
URL | https://pubchem.ncbi.nlm.nih.gov | |
Description | Data deposited in or computed by PubChem | |
Molecular Formula |
C6H12O6 | |
Details | Computed by PubChem 2.1 (PubChem release 2021.05.07) | |
Source | PubChem | |
URL | https://pubchem.ncbi.nlm.nih.gov | |
Description | Data deposited in or computed by PubChem | |
DSSTOX Substance ID |
DTXSID80858960 | |
Record name | Hexopyranose | |
Source | EPA DSSTox | |
URL | https://comptox.epa.gov/dashboard/DTXSID80858960 | |
Description | DSSTox provides a high quality public chemistry resource for supporting improved predictive toxicology. | |
Molecular Weight |
180.16 g/mol | |
Details | Computed by PubChem 2.1 (PubChem release 2021.05.07) | |
Source | PubChem | |
URL | https://pubchem.ncbi.nlm.nih.gov | |
Description | Data deposited in or computed by PubChem | |
CAS RN |
42752-07-8, 579-36-2, 2280-44-6, 39392-65-9, 10257-28-0 | |
Record name | Hexopyranose | |
Source | CAS Common Chemistry | |
URL | https://commonchemistry.cas.org/detail?cas_rn=42752-07-8 | |
Description | CAS Common Chemistry is an open community resource for accessing chemical information. Nearly 500,000 chemical substances from CAS REGISTRY cover areas of community interest, including common and frequently regulated chemicals, and those relevant to high school and undergraduate chemistry classes. This chemical information, curated by our expert scientists, is provided in alignment with our mission as a division of the American Chemical Society. | |
Explanation | The data from CAS Common Chemistry is provided under a CC-BY-NC 4.0 license, unless otherwise stated. | |
Record name | D-Allopyranose | |
Source | DTP/NCI | |
URL | https://dtp.cancer.gov/dtpstandard/servlet/dwindex?searchtype=NSC&outputformat=html&searchlist=144657 | |
Description | The NCI Development Therapeutics Program (DTP) provides services and resources to the academic and private-sector research communities worldwide to facilitate the discovery and development of new cancer therapeutic agents. | |
Explanation | Unless otherwise indicated, all text within NCI products is free of copyright and may be reused without our permission. Credit the National Cancer Institute as the source. | |
Record name | NSC287045 | |
Source | DTP/NCI | |
URL | https://dtp.cancer.gov/dtpstandard/servlet/dwindex?searchtype=NSC&outputformat=html&searchlist=287045 | |
Description | The NCI Development Therapeutics Program (DTP) provides services and resources to the academic and private-sector research communities worldwide to facilitate the discovery and development of new cancer therapeutic agents. | |
Explanation | Unless otherwise indicated, all text within NCI products is free of copyright and may be reused without our permission. Credit the National Cancer Institute as the source. | |
Record name | NSC274237 | |
Source | DTP/NCI | |
URL | https://dtp.cancer.gov/dtpstandard/servlet/dwindex?searchtype=NSC&outputformat=html&searchlist=274237 | |
Description | The NCI Development Therapeutics Program (DTP) provides services and resources to the academic and private-sector research communities worldwide to facilitate the discovery and development of new cancer therapeutic agents. | |
Explanation | Unless otherwise indicated, all text within NCI products is free of copyright and may be reused without our permission. Credit the National Cancer Institute as the source. | |
Record name | D-galactose | |
Source | DTP/NCI | |
URL | https://dtp.cancer.gov/dtpstandard/servlet/dwindex?searchtype=NSC&outputformat=html&searchlist=8102 | |
Description | The NCI Development Therapeutics Program (DTP) provides services and resources to the academic and private-sector research communities worldwide to facilitate the discovery and development of new cancer therapeutic agents. | |
Explanation | Unless otherwise indicated, all text within NCI products is free of copyright and may be reused without our permission. Credit the National Cancer Institute as the source. | |
Record name | Hexopyranose | |
Source | EPA DSSTox | |
URL | https://comptox.epa.gov/dashboard/DTXSID80858960 | |
Description | DSSTox provides a high quality public chemistry resource for supporting improved predictive toxicology. | |
Synthesis routes and methods I
Procedure details
Synthesis routes and methods II
Procedure details
体外研究产品的免责声明和信息
请注意,BenchChem 上展示的所有文章和产品信息仅供信息参考。 BenchChem 上可购买的产品专为体外研究设计,这些研究在生物体外进行。体外研究,源自拉丁语 "in glass",涉及在受控实验室环境中使用细胞或组织进行的实验。重要的是要注意,这些产品没有被归类为药物或药品,他们没有得到 FDA 的批准,用于预防、治疗或治愈任何医疗状况、疾病或疾病。我们必须强调,将这些产品以任何形式引入人类或动物的身体都是法律严格禁止的。遵守这些指南对确保研究和实验的法律和道德标准的符合性至关重要。