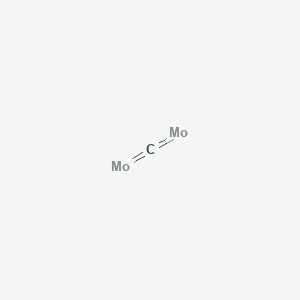
Molybdenum carbide
- 専門家チームからの見積もりを受け取るには、QUICK INQUIRYをクリックしてください。
- 品質商品を競争力のある価格で提供し、研究に集中できます。
説明
Molybdenum carbide is an extremely hard, refractory, ceramic material, commercially used in tool bits for cutting tools . It has unique physicochemical properties such as high mechanical hardness, thermal stability, high melting point, resistance to mechanical and corrosive wear, and good thermal and electrical conductivity .
Synthesis Analysis
This compound can be synthesized using a carbothermal hydrogen reduction method . A simple solid mixture of (NH4)6Mo7O24·4H2O and carbon nanotubes (CNTs) can be used as a precursor . The carburization degree can be controlled by selecting different carburization temperatures .
Molecular Structure Analysis
Molybdenum carbides have multiple crystal structures such as α-MoC, δ-MoC, β-Mo2C with C-terminated, Mo-terminated or C/Mo mixed terminated surfaces . The crystal structures of transition metal compounds with carbon can be described as the closest packing of metal atoms with smaller carbon atoms inserted in the interstices .
Chemical Reactions Analysis
This compound displays promising catalytic performance in reactions such as alkane hydrogenolysis, hydrodenitrogenation of organonitrogen compounds, dry reforming of methane, the water–gas shift (WGS) reaction, and CO hydrogenation . It also shows excellent catalytic performance in the hydrogenation of carbon dioxide .
Physical And Chemical Properties Analysis
This compound has unique physicochemical properties: high mechanical hardness, thermal stability, high melting point, resistance to mechanical and corrosive wear, and good thermal and electrical conductivity .
科学的研究の応用
Photocatalysis Applications : Molybdenum carbide is noted for its excellent biocompatibility, high stability, adjustable band gap, rich valence states, and catalytic activity, making it a promising material in photocatalysis. Various strategies like doping engineering, vacancy engineering, and morphology control have been employed to enhance its photocatalytic performance (Zhang, Wang, Guo, & Wang, 2022).
Electrocatalysis for Hydrogen Evolution Reaction (HER) : Iron-doped this compound demonstrates high activity and stability as a catalyst for HER, a promising alternative to platinum. The addition of magnetic iron dopants significantly enhances its catalytic activity (Wan & Leonard, 2015).
Optoelectronic and Photonic Applications : this compound (Mo2C) shows potential in optoelectronic and photonic fields due to its electrical and optical characteristics. Its use in Q-switched laser applications at 639 nm demonstrates impressive nonlinear optical properties (Liu et al., 2022).
Energy Conversion and Electrochemical Applications : Porous this compound nanostructures have been synthesized for efficient hydrogen evolution, highlighting its role in energy conversion and electrocatalysis. These structures show low onset overpotential and great stability (Wan et al., 2017).
Electrochemical Stability Analysis : A study comparing the electrochemical stability of transition metal carbides, including this compound, across a wide pH range, highlights its potential as a low-cost catalyst or catalyst support material in electrochemical applications (Weidman, Esposito, Hsu, & Chen, 2012).
作用機序
Target of Action
Molybdenum carbide (MFCD00014218) is primarily used as a catalyst in various chemical reactions . It is particularly instrumental in the physical vapor deposition (PVD) process, which is critical for applying thin film coatings on various substrates . These substrates find applications across a multitude of industries .
Mode of Action
This compound interacts with its targets through a process known as sputtering. In this process, molybdenum atoms are sputtered from the target’s surface through ion bombardment and subsequently deposited onto the substrate . This process is critical for the application of thin film coatings on various substrates .
Biochemical Pathways
This compound has been suggested as a catalyst for carbon dioxide hydrogenation . It has also been used in the redox reactions of organic compounds . The attention of researchers is focused on the use of this compound as a catalyst for hydrogen production in the following main reactions: dry methane reforming, steam reforming of methanol, water gas shift reaction (WGSR), and hydrogen evolution by electrolysis of water .
Pharmacokinetics
This compound is an extremely hard, refractory, ceramic material . It is insoluble in water , which suggests that it would have low bioavailability if ingested or inhaled.
Result of Action
The molecular and cellular effects of this compound’s action are largely dependent on its role as a catalyst. For example, in carbon dioxide hydrogenation, this compound facilitates the conversion of CO2 into other compounds . In the realm of material science and thin-film technology, this compound helps in the deposition of thin films on various substrates .
Action Environment
The efficacy and stability of this compound can be influenced by various environmental factors. For instance, the preparation methods of this compound can affect its crystal structure and catalytic activity . Furthermore, sulfur-doping, Ni-doping, and heterophase structure have been highlighted as important factors for enhancing the hydrogen evolution reaction (HER) activity of this compound .
将来の方向性
Molybdenum carbide has attracted extensive attention in the field of sustainable hydrogen production due to its excellent Pt-like catalytic activity, low cost, high chemical stability, and natural abundance . Future research directions include optimizing the catalytic activity of MoxC, including optimization of synthesis methods, composites with carbon material, non-precious metal doping, transition metal doping, construction of the heterogeneous structure, etc .
特性
InChI |
InChI=1S/C.2Mo |
Source
|
---|---|---|
Details | Computed by InChI 1.0.5 (PubChem release 2019.06.18) | |
Source | PubChem | |
URL | https://pubchem.ncbi.nlm.nih.gov | |
Description | Data deposited in or computed by PubChem | |
InChI Key |
QIJNJJZPYXGIQM-UHFFFAOYSA-N |
Source
|
Details | Computed by InChI 1.0.5 (PubChem release 2019.06.18) | |
Source | PubChem | |
URL | https://pubchem.ncbi.nlm.nih.gov | |
Description | Data deposited in or computed by PubChem | |
Canonical SMILES |
C(=[Mo])=[Mo] |
Source
|
Details | Computed by OEChem 2.1.5 (PubChem release 2019.06.18) | |
Source | PubChem | |
URL | https://pubchem.ncbi.nlm.nih.gov | |
Description | Data deposited in or computed by PubChem | |
Molecular Formula |
CMo2 |
Source
|
Details | Computed by PubChem 2.1 (PubChem release 2019.06.18) | |
Source | PubChem | |
URL | https://pubchem.ncbi.nlm.nih.gov | |
Description | Data deposited in or computed by PubChem | |
Molecular Weight |
203.9 g/mol |
Source
|
Details | Computed by PubChem 2.1 (PubChem release 2021.05.07) | |
Source | PubChem | |
URL | https://pubchem.ncbi.nlm.nih.gov | |
Description | Data deposited in or computed by PubChem | |
Q & A
Q1: What are the common crystal structures of molybdenum carbide?
A1: this compound exists in various crystal phases, including hexagonal close-packed (hcp) β-Mo2C, face-centered cubic (fcc) α-MoC1-x, and η-Mo3C2. The specific phase obtained depends on the synthesis method and conditions. [, , , ]
Q2: How does the choice of the carbon source during synthesis affect the properties of this compound?
A2: The carbon source significantly influences the structure and texture of the resulting this compound. Using longer chain hydrocarbons as the carburizing agent generally leads to smaller particle size and lower temperatures required for complete transformation from molybdenum oxide to carbide. For instance, using methane tends to yield hcp carbide, while butane favors fcc carbide. []
Q3: How does the surface area of this compound impact its catalytic activity?
A3: High surface area this compound typically exhibits enhanced catalytic activity due to the increased availability of active sites. Supporting this compound on materials like alumina or carbon nanotubes can help achieve higher surface areas and improve catalyst dispersion. [, , , ]
Q4: What are the advantages of using this compound in hydrodesulfurization (HDS) reactions?
A4: this compound displays promising activity in HDS reactions, showing resistance to poisoning by sulfur compounds. This is advantageous compared to some other catalysts that are readily deactivated by sulfur. [, ]
Q5: What role does cobalt play in enhancing the catalytic activity of this compound for thiophene HDS?
A5: Doping this compound with cobalt can significantly enhance its activity for thiophene HDS. This improvement is attributed to factors like reduced carburizing temperature, smaller particle size, increased surface area, and higher Mo2+ content on the catalyst surface. The optimal Co/Mo molar ratio for maximum synergistic interaction is around 0.2. []
Q6: How does this compound compare to noble metal catalysts in the conversion of vegetable oils?
A6: this compound catalysts have shown promising activity and selectivity in the hydrodeoxygenation of vegetable oils, converting them into diesel-like hydrocarbons. They exhibit good resistance to leaching compared to noble metal catalysts, making them potentially more cost-effective and sustainable for this application. [, ]
Q7: Can this compound catalyze the hydrogen evolution reaction (HER)?
A7: Yes, this compound has garnered significant interest as an electrocatalyst for HER due to its Pt-like electronic properties. Various strategies like phase-transition engineering, heteroatom doping, and construction of heterostructures have been explored to enhance its HER activity. [, , ]
Q8: How does the presence of surface molybdenum oxide impact the performance of this compound in HER?
A8: Surface molybdenum oxide, often formed due to air exposure, can hinder the performance of this compound in HER. In situ removal of this oxide layer during catalyst preparation can significantly enhance the catalytic activity. []
Q9: What are the common methods for synthesizing this compound?
A9: Several methods exist for this compound synthesis, including:
- Temperature-programmed reaction (TPR): This method involves the controlled carburization of molybdenum oxide precursors (e.g., MoO3) using a carburizing gas mixture like CH4/H2. [, , , , ]
- Carbothermal reduction: This method utilizes a solid carbon source to reduce and carburize molybdenum oxide at high temperatures. [, ]
- Electrolysis: this compound can be prepared through the co-electrolysis of precursors like Na2MoO4 and CO2 in molten salt electrolytes. []
- Microwave-induced synthesis: This method utilizes microwave irradiation to facilitate the rapid and energy-efficient synthesis of this compound. [, ]
- DC arc plasma: This method employs a high-temperature plasma generated by a DC arc discharge to synthesize this compound nanoparticles, often embedded in a carbon matrix. [, ]
Q10: How can the crystal phase of this compound be controlled during synthesis?
A10: The crystal phase of this compound can be controlled by adjusting synthesis parameters such as:
- Temperature: Different phases are thermodynamically favored at different temperatures. [, ]
- Carburizing agent: The choice of the hydrocarbon used during carburization influences the resulting phase. []
- Precursor and support: The nature of the molybdenum precursor and the support material can also influence the phase formation. [, ]
Q11: What are the common characterization techniques used to study this compound?
A11: Various techniques are employed to characterize this compound materials, including:
- X-ray diffraction (XRD): Used to identify the crystal structure and phase purity of the material. [, , , , , , ]
- Scanning electron microscopy (SEM) and Transmission electron microscopy (TEM): Provide information about the morphology and particle size of the material. [, , , , , , , ]
- X-ray photoelectron spectroscopy (XPS): Used to determine the elemental composition and chemical states of elements present in the material. [, , , , ]
- Nitrogen adsorption-desorption analysis: Provides information about the surface area and porosity of the material. [, , , , , ]
- Raman spectroscopy: Can be used to characterize the carbon structure in this compound materials. [, ]
Q12: What is the role of temperature in the formation of this compound during TPR?
A12: Temperature plays a crucial role in the stepwise transformation of molybdenum oxide to carbide during TPR. The reaction often proceeds through intermediate phases like MoO2 and MoOxCy before forming the desired this compound phase. For example, the optimal carburizing temperature for forming β-Mo2C from MoO3 using CH4/H2 is around 675 °C. []
Q13: How is density functional theory (DFT) used to study this compound?
A13: DFT calculations are widely employed to investigate various aspects of this compound, such as:
- Electronic structure: DFT can elucidate the electronic density of states, band structure, and bonding characteristics of different this compound phases. [, ]
- Surface properties: DFT calculations provide insights into the adsorption energies of reactants and intermediates on different this compound surfaces, helping understand reaction mechanisms. [, , ]
- Reaction mechanisms: DFT can be used to study the elementary steps involved in catalytic reactions on this compound surfaces, identifying reaction pathways and transition states. []
Q14: Can you provide an example of how DFT has been used to understand a specific reaction on this compound?
A14: DFT calculations have been employed to investigate the water-gas shift (WGS) reaction mechanism on this compound surfaces. The calculations revealed the adsorption energies of water, carbon monoxide, and reaction intermediates on different surface sites. The results suggested a mechanism involving water dissociation followed by CO oxidation to CO2. []
Q15: How can DFT simulations help in designing more efficient this compound-based catalysts?
A15: DFT simulations provide valuable insights into the structure-activity relationships of this compound catalysts. By modeling the effects of factors like doping, surface modifications, and support interactions, researchers can predict how these modifications influence catalytic activity and selectivity. This knowledge can guide the rational design of more efficient and selective this compound-based catalysts.
Q16: What are the stability concerns associated with this compound catalysts?
A16: While this compound exhibits good resistance to sulfur poisoning compared to some catalysts, one major stability concern is its susceptibility to oxidation under certain conditions. This can lead to the formation of molybdenum oxide, which can negatively impact its catalytic performance. [, ]
Q17: How can the stability of this compound catalysts be improved?
A17: Several strategies can be employed to enhance the stability of this compound catalysts:
- Controlling the synthesis conditions: Optimizing the carburization process during synthesis can lead to the formation of more stable this compound phases. [, , ]
- Adding promoters or dopants: Incorporating elements like nickel or cobalt can improve the stability of this compound catalysts. [, ]
- Using suitable supports: Supporting this compound on materials like alumina, carbon nanotubes, or porous silica can enhance its stability and prevent sintering. [, , , ]
- Passivating the surface: Controlled surface passivation with a thin layer of a protective material can prevent oxidation and improve stability. []
Q18: What are the environmental benefits of using this compound catalysts?
A18: this compound catalysts offer several environmental advantages:
- Reduced reliance on noble metals: They provide a cost-effective and sustainable alternative to expensive and less abundant noble metal catalysts like platinum. [, ]
- Potential for biomass conversion: this compound catalysts have shown promise in converting renewable biomass feedstocks into valuable chemicals and fuels. [, , ]
- CO2 utilization: Electrolysis methods for synthesizing this compound can potentially utilize CO2 as a carbon source, contributing to greenhouse gas mitigation. []
試験管内研究製品の免責事項と情報
BenchChemで提示されるすべての記事および製品情報は、情報提供を目的としています。BenchChemで購入可能な製品は、生体外研究のために特別に設計されています。生体外研究は、ラテン語の "in glass" に由来し、生物体の外で行われる実験を指します。これらの製品は医薬品または薬として分類されておらず、FDAから任何の医療状態、病気、または疾患の予防、治療、または治癒のために承認されていません。これらの製品を人間または動物に体内に導入する形態は、法律により厳格に禁止されています。これらのガイドラインに従うことは、研究と実験において法的および倫理的な基準の遵守を確実にするために重要です。