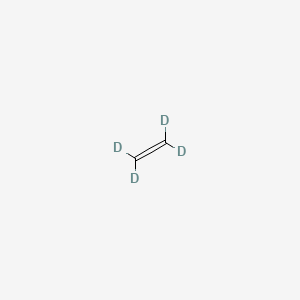
(2H4)Ethylene
概要
説明
(2H4)Ethylene, also known as C2D4, is a variant of Ethylene where the hydrogen atoms are replaced by deuterium . Ethylene, with the formula C2H4, is a crucial compound in the chemical industry and is often used in polymer production .
Synthesis Analysis
Ethylene is a plant hormone involved in many aspects of the plant life cycle, including seed germination, root hair development, flower senescence, abscission, and fruit ripening . The production of ethylene is tightly regulated by internal signals during development and in response to environmental stimuli from biotic and abiotic stresses .Molecular Structure Analysis
Ethylene is an unsaturated organic compound with a Carbon-Carbon double bond . Each carbon atom of ethylene is sp2 hybridized . The molecular weight of this compound is 32.0778 .Chemical Reactions Analysis
The chemical reactions of this compound can be studied through its ion energetics data . The ionization energy of this compound has been determined to be 10.528 ± 0.001 eV .Physical And Chemical Properties Analysis
This compound is a gas under normal conditions . The molecular weight of this compound is 32.0778 . Ethylene is a colorless gas which has a sweet odor and taste .科学的研究の応用
Ethane/Ethylene Separation Ethylene, a critical chemical used as a feedstock in the artificial plastic chemistry fields, is often accompanied by ethane in the oil cracking process. Research shows that Ni(IN)2, a metal–organic framework (MOF), is a promising adsorbent for ethane/ethylene separation, demonstrating high selectivity and excellent recyclability. This material is beneficial for producing high-purity C2H4 in a single step through adsorptive separation (Kang et al., 2021).
Combustion Chemistry Ethylene is an important intermediate in the oxidation and pyrolysis of higher hydrocarbons and is used as a fuel in practical engines. Studies involving shock tube experiments and simulations have explored the combustion chemistry of ethylene–air mixtures under a wide range of conditions. This research is crucial for understanding ignition delay times and the influence of ethylene on combustion processes (Yang et al., 2020).
Ethane Dehydrogenation With the increasing demand for ethylene, research has focused on the development of new processes to reduce energy consumption in its production. The ethane dehydrogenation (EDH) reaction in microporous zeolite membrane reactors is an attractive solution for enhancing ethane conversion and ethylene yield, surpassing the equilibrium limit of ethane conversion at high temperatures (Dangwal, Liu, & Kim, 2017).
Safety and Hazard Assessment Ethylene is highly flammable, presenting a serious fire and explosion hazard. Research using large eddy simulation (LES) method has addressed the hazard assessment of ethylene mixtures in different scale channels, studying flame acceleration and deflagration-to-detonation transition. This is crucial for ensuring safety in industries where ethylene is used (Wang, Zhao, & Zhang, 2016).
Horticultural Applications Ethylene acts as a plant hormone, affecting many aspects of plant growth and development. Research has shown that the etr1-1 gene in Arabidopsis encodes a mutated receptor that confers dominant ethylene insensitivity, affecting fruit ripening, flower senescence, and abscission in heterologous plants like tomato and petunia. This suggests the possibility of manipulating ethylene perception pathways in various plants for agricultural purposes (Wilkinson et al., 1997).
- tomatoes. This degradation is important for reducing the harmful effects of ethylene during postharvest handling of crops, such as accelerating senescence and browning of vegetal tissues. The research found that a specific TiO2/SiO2 nanocomposite showed the best degradation activity, resulting in complete ethylene removal, which is beneficial for prolonging the shelf life of fresh produce like tomatoes (De Chiara et al., 2015).
作用機序
Target of Action
Ethylene-d4, also known as (2H4)Ethylene, primarily targets ethylene receptors in plants . These receptors play a crucial role in many aspects of plant growth, development, and stress responses . Ethylene signaling is initiated upon its binding to the ethylene receptors .
Mode of Action
Ethylene-d4 interacts with its targets, the ethylene receptors, resulting in various changes in plant physiology . The binding of ethylene to the receptors triggers a series of responses and effects observed in seed germination, stem cell division, cell elongation and differentiation, root hair growth, seedling nutation, sex determination, fruit ripening, senescence, abscission, and responses to salt, drought, flooding, cold stresses, etc .
Biochemical Pathways
Ethylene-d4 affects several biochemical pathways. It is synthesized from S-adenosylmethionine (SAM) via 1-aminocyclopropane-1-carboxylic acid (ACC). In plants, ACC synthase (ACS) and ACC oxidase (ACO), two key enzymes in the ethylene biosynthetic pathway, are tightly regulated both transcriptionally and posttranscriptionally to modulate ethylene biosynthesis . The ethylene-induced ETR2 is reduced in etr1 ethylene-insensitive, gain of function mutants, and elevated in etr1 loss-of-function mutants, indicating that ethylene-induced transcription of receptor gene is also regulated by other ethylene receptors .
Result of Action
The molecular and cellular effects of Ethylene-d4’s action are diverse. It plays a crucial role in regulating plant signaling networks involved in responding to multiple biotic and abiotic stresses . It influences performance of plants under optimal and stressful environments by interacting with other signaling molecules . The action of ethylene depends on its concentration in the cell and the sensitivity of plants to the hormone .
Action Environment
Environmental factors significantly influence the action, efficacy, and stability of Ethylene-d4. Ethylene production is upregulated in response to diverse biotic and abiotic stressors such as pathogen attack, wounding, dehydration, chilling, and ozone . The lack of mobility in plants resulted in a complicated process of adaptation to the environment and the acquisition of defense mechanisms against diseases and predators .
Safety and Hazards
将来の方向性
The future of ethylene research is promising, with a focus on regulatory networks controlling ethylene synthesis and its role in flower development and fruit ripening . There is also interest in the electrochemical reduction of carbon dioxide to ethylene, which could provide a promising way to convert CO2 to chemicals .
生化学分析
Biochemical Properties
Ethylene-d4, like its parent molecule ethylene, interacts with a variety of enzymes and proteins. The ethylene signaling pathway is initiated upon its binding to the ethylene receptors . Ethylene-d4 may also interact with these receptors, potentially influencing biochemical reactions in a similar manner to ethylene.
Cellular Effects
Ethylene-d4, due to its similarity to ethylene, may influence various types of cells and cellular processes. Ethylene has been shown to affect seed germination, stem cell division, cell elongation and differentiation, root hair growth, and more . It’s plausible that Ethylene-d4 could have similar effects on cell function, including impacts on cell signaling pathways, gene expression, and cellular metabolism.
Molecular Mechanism
The molecular mechanism of Ethylene-d4 is likely to be similar to that of ethylene. Ethylene exerts its effects at the molecular level through binding interactions with biomolecules, enzyme inhibition or activation, and changes in gene expression . Ethylene-d4, due to its structural similarity, may interact with the same biomolecules and exert similar effects.
Temporal Effects in Laboratory Settings
Ethylene has been shown to have temporal effects on plant growth and development . It’s possible that Ethylene-d4 could have similar temporal effects, including impacts on the product’s stability, degradation, and long-term effects on cellular function.
Metabolic Pathways
Ethylene-d4 is likely involved in similar metabolic pathways as ethylene. Ethylene is produced in most tissues and cell types in higher plants, and its biosynthesis is tightly regulated . Ethylene-d4, due to its structural similarity, may be involved in similar metabolic pathways.
Transport and Distribution
Ethylene is a gaseous molecule, which can be freely transported from one cell to another by diffusion . Given its similar structure, Ethylene-d4 is likely to have similar transport and distribution properties within cells and tissues.
Subcellular Localization
The subcellular localization of Ethylene-d4 is likely to be similar to that of ethylene. Most components of the ethylene signaling pathway, other than transcription factors and protein turnover machinery, are associated with or lie within the endoplasmic reticulum (ER) membrane . Therefore, Ethylene-d4 may also be associated with the ER membrane.
特性
IUPAC Name |
1,1,2,2-tetradeuterioethene | |
---|---|---|
Source | PubChem | |
URL | https://pubchem.ncbi.nlm.nih.gov | |
Description | Data deposited in or computed by PubChem | |
InChI |
InChI=1S/C2H4/c1-2/h1-2H2/i1D2,2D2 | |
Source | PubChem | |
URL | https://pubchem.ncbi.nlm.nih.gov | |
Description | Data deposited in or computed by PubChem | |
InChI Key |
VGGSQFUCUMXWEO-LNLMKGTHSA-N | |
Source | PubChem | |
URL | https://pubchem.ncbi.nlm.nih.gov | |
Description | Data deposited in or computed by PubChem | |
Canonical SMILES |
C=C | |
Source | PubChem | |
URL | https://pubchem.ncbi.nlm.nih.gov | |
Description | Data deposited in or computed by PubChem | |
Isomeric SMILES |
[2H]C(=C([2H])[2H])[2H] | |
Source | PubChem | |
URL | https://pubchem.ncbi.nlm.nih.gov | |
Description | Data deposited in or computed by PubChem | |
Molecular Formula |
C2H4 | |
Source | PubChem | |
URL | https://pubchem.ncbi.nlm.nih.gov | |
Description | Data deposited in or computed by PubChem | |
Related CAS |
25549-98-8 | |
Record name | Ethene-1,1,2,2-d4, homopolymer | |
Source | CAS Common Chemistry | |
URL | https://commonchemistry.cas.org/detail?cas_rn=25549-98-8 | |
Description | CAS Common Chemistry is an open community resource for accessing chemical information. Nearly 500,000 chemical substances from CAS REGISTRY cover areas of community interest, including common and frequently regulated chemicals, and those relevant to high school and undergraduate chemistry classes. This chemical information, curated by our expert scientists, is provided in alignment with our mission as a division of the American Chemical Society. | |
Explanation | The data from CAS Common Chemistry is provided under a CC-BY-NC 4.0 license, unless otherwise stated. | |
DSSTOX Substance ID |
DTXSID30218454 | |
Record name | (2H4)Ethylene | |
Source | EPA DSSTox | |
URL | https://comptox.epa.gov/dashboard/DTXSID30218454 | |
Description | DSSTox provides a high quality public chemistry resource for supporting improved predictive toxicology. | |
Molecular Weight |
32.08 g/mol | |
Source | PubChem | |
URL | https://pubchem.ncbi.nlm.nih.gov | |
Description | Data deposited in or computed by PubChem | |
CAS RN |
683-73-8 | |
Record name | Ethene-d4 | |
Source | CAS Common Chemistry | |
URL | https://commonchemistry.cas.org/detail?cas_rn=683-73-8 | |
Description | CAS Common Chemistry is an open community resource for accessing chemical information. Nearly 500,000 chemical substances from CAS REGISTRY cover areas of community interest, including common and frequently regulated chemicals, and those relevant to high school and undergraduate chemistry classes. This chemical information, curated by our expert scientists, is provided in alignment with our mission as a division of the American Chemical Society. | |
Explanation | The data from CAS Common Chemistry is provided under a CC-BY-NC 4.0 license, unless otherwise stated. | |
Record name | (2H4)Ethylene | |
Source | ChemIDplus | |
URL | https://pubchem.ncbi.nlm.nih.gov/substance/?source=chemidplus&sourceid=0000683738 | |
Description | ChemIDplus is a free, web search system that provides access to the structure and nomenclature authority files used for the identification of chemical substances cited in National Library of Medicine (NLM) databases, including the TOXNET system. | |
Record name | (2H4)Ethylene | |
Source | EPA DSSTox | |
URL | https://comptox.epa.gov/dashboard/DTXSID30218454 | |
Description | DSSTox provides a high quality public chemistry resource for supporting improved predictive toxicology. | |
Record name | [2H4]ethylene | |
Source | European Chemicals Agency (ECHA) | |
URL | https://echa.europa.eu/substance-information/-/substanceinfo/100.010.615 | |
Description | The European Chemicals Agency (ECHA) is an agency of the European Union which is the driving force among regulatory authorities in implementing the EU's groundbreaking chemicals legislation for the benefit of human health and the environment as well as for innovation and competitiveness. | |
Explanation | Use of the information, documents and data from the ECHA website is subject to the terms and conditions of this Legal Notice, and subject to other binding limitations provided for under applicable law, the information, documents and data made available on the ECHA website may be reproduced, distributed and/or used, totally or in part, for non-commercial purposes provided that ECHA is acknowledged as the source: "Source: European Chemicals Agency, http://echa.europa.eu/". Such acknowledgement must be included in each copy of the material. ECHA permits and encourages organisations and individuals to create links to the ECHA website under the following cumulative conditions: Links can only be made to webpages that provide a link to the Legal Notice page. | |
Synthesis routes and methods I
Procedure details
Synthesis routes and methods II
Procedure details
Synthesis routes and methods III
Procedure details
Synthesis routes and methods IV
Procedure details
Retrosynthesis Analysis
AI-Powered Synthesis Planning: Our tool employs the Template_relevance Pistachio, Template_relevance Bkms_metabolic, Template_relevance Pistachio_ringbreaker, Template_relevance Reaxys, Template_relevance Reaxys_biocatalysis model, leveraging a vast database of chemical reactions to predict feasible synthetic routes.
One-Step Synthesis Focus: Specifically designed for one-step synthesis, it provides concise and direct routes for your target compounds, streamlining the synthesis process.
Accurate Predictions: Utilizing the extensive PISTACHIO, BKMS_METABOLIC, PISTACHIO_RINGBREAKER, REAXYS, REAXYS_BIOCATALYSIS database, our tool offers high-accuracy predictions, reflecting the latest in chemical research and data.
Strategy Settings
Precursor scoring | Relevance Heuristic |
---|---|
Min. plausibility | 0.01 |
Model | Template_relevance |
Template Set | Pistachio/Bkms_metabolic/Pistachio_ringbreaker/Reaxys/Reaxys_biocatalysis |
Top-N result to add to graph | 6 |
Feasible Synthetic Routes
Q & A
Q1: What is the molecular formula and weight of ethylene-d4?
A1: Ethylene-d4 has the molecular formula C2D4. Its molecular weight is 32.10 g/mol.
Q2: How is the structure of ethylene-d4 confirmed?
A2: Various spectroscopic techniques are employed to characterize ethylene-d4, including Nuclear Magnetic Resonance (NMR) spectroscopy and Infrared (IR) spectroscopy. For example, [] utilizes 13C NMR to analyze copolymers containing ethylene-d4. [https://www.semanticscholar.org/paper/d634fed8f82cdcbf5a893929c1c2598b66807b61]
Q3: What are the key vibrational modes observed in ethylene-d4?
A3: Infrared and Raman spectroscopy reveal significant vibrational modes in ethylene-d4, including the ν9 fundamental band around 2341 cm−1 [] and the ν11 band around 2201 cm−1 []. [https://www.semanticscholar.org/paper/5cfa7db7f0a3ba330c97366d67699acb328540b6] [https://www.semanticscholar.org/paper/0bca86ef46d1aeb77eb8bb96b60aa3a19c588c4e]
Q4: How does ethylene-d4 behave in a liquid nitrogen solution?
A4: [] studied the reaction of ethylene-d4 with oxygen atoms in liquid nitrogen solution at 77°K. The primary product observed was ethylene oxide, demonstrating the reactivity of ethylene-d4 in cryogenic conditions. [https://www.semanticscholar.org/paper/486a876a37f679a2122e3c90dc29516cfe6c9835]
Q5: Can ethylene-d4 be incorporated into polymers?
A5: Yes, ethylene-d4 can be copolymerized with other monomers like ethylene and propylene. [] describes the synthesis and characterization of homogeneous copolymers of ethylene-d4 and 1-alkenes using specific catalyst systems. [https://www.semanticscholar.org/paper/c9bd15325962726b7985eaf13f88659a074393be]
Q6: How is ethylene-d4 used in studying catalytic reactions?
A6: Ethylene-d4 serves as a valuable isotopic label to elucidate reaction mechanisms in catalysis. For instance, [] uses ethylene-d4 to investigate the activation mechanism of bimetallic precatalysts in norbornene polymerization. [https://www.semanticscholar.org/paper/a8b8cbca9ce65c8fbf25a0f0eb9a5cc7b0f52bfd]
Q7: Does the use of ethylene-d4 instead of ethylene affect polymerization reactions?
A7: Yes, isotopic substitution can impact polymerization kinetics. [] showed that deuterium, as present in ethylene-d4, decreases the reactivity of ethylene in Ziegler-Natta catalyzed polymerization. [https://www.semanticscholar.org/paper/fccd1c6daec4bc1084170e89b8a177f4866578d9]
Q8: How is computational chemistry used to study ethylene-d4?
A8: Density Functional Theory (DFT) calculations are utilized to study the properties and reactivity of ethylene-d4. [] employed DFT to investigate the equilibrium deuterium isotope effect in the electrophilic bromination of ethylene and ethylene-d4. [https://www.semanticscholar.org/paper/acaa899c02ddf4b08b94177c13be1e522e8200ce]
Q9: Can computational models predict the behavior of ethylene-d4 in chemical reactions?
A9: Yes, theoretical calculations, including those based on the Rice-Ramsperger-Kassel-Marcus (RRKM) theory, can help predict the reaction dynamics of ethylene-d4. [] combined experimental data with ab initio calculations and RRKM theory to understand the reaction of the 1-propynyl radical with ethylene and ethylene-d4. [https://www.semanticscholar.org/paper/94b3d2e1364bf16c3e4207dc31ee235988825067]
試験管内研究製品の免責事項と情報
BenchChemで提示されるすべての記事および製品情報は、情報提供を目的としています。BenchChemで購入可能な製品は、生体外研究のために特別に設計されています。生体外研究は、ラテン語の "in glass" に由来し、生物体の外で行われる実験を指します。これらの製品は医薬品または薬として分類されておらず、FDAから任何の医療状態、病気、または疾患の予防、治療、または治癒のために承認されていません。これらの製品を人間または動物に体内に導入する形態は、法律により厳格に禁止されています。これらのガイドラインに従うことは、研究と実験において法的および倫理的な基準の遵守を確実にするために重要です。