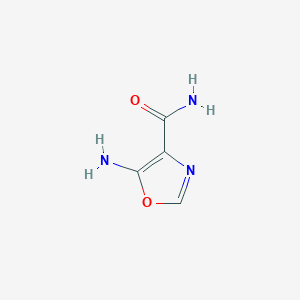
5-Aminooxazole-4-carboxamide
概要
説明
5-Aminooxazole-4-carboxamide is a significant small-molecule scaffold that has gained increasing attention in the field of chemical biology. It is a natural metabolic intermediate of purine biosynthesis present in all organisms .
Synthesis Analysis
The synthesis of this compound has been reported in various studies. For instance, an efficient synthesis of 5-amino-1H-imidazole-4-carboxamide (AIC) from commercially available hypoxanthine was described . Another study reported a new synthesis method with a high total yield (79%) of 5-aminothiazole-4-carboxamide .Molecular Structure Analysis
The molecular formula of this compound is C4H5N3O2 . It is an aminoimidazole in which the amino group is at C-5 with a carboxamido group at C-4 .科学的研究の応用
Synthesis and Chemical Properties
- Microwave-Assisted Preparation : A study by Nolt et al. (2006) optimized the preparation of oxazole-4-carboxamides, leading to 5-aminooxazole-4-carboxylates through a microwave-assisted procedure. This method proved effective with a variety of substituted oxazoles, yielding products of high purity and good yield (Nolt et al., 2006).
Application in Drug Development and Biological Studies
- Inhibitor Development : A study by Zhang et al. (2014) utilized 5-aminopyrazole-4-carboxamide as a scaffold to develop selective inhibitors of calcium-dependent protein kinase-1 in Toxoplasma gondii and Cryptosporidium parvum. This led to the creation of compounds with significant inhibitory potencies against the target enzymes (Zhang et al., 2014).
Synthesis Methods and Chemical Transformations
- Innovative Synthesis Techniques : A study by Sekiya and Suzuki (1970) discovered a new method for preparing a series of 5-acylaminooxazole-4-carboxamides, expanding the possibilities for synthesizing oxazolo[5, 4-d]pyrimidines (Sekiya & Suzuki, 1970).
- Auxiliary-Directed Activation : Pasunooti et al. (2015) explored bidentate auxiliaries derived from isoxazole-3-carboxamide and oxazole-4-carboxamide moieties for Pd-catalyzed C(sp(3))-H bond activation. This enabled the selective and efficient synthesis of various γ-substituted non-natural amino acids (Pasunooti et al., 2015).
Biological Mechanisms and Interactions
- Mechanism of Action Studies : Saunders and Chao (1974) investigated the mechanism of action of 5-(3,3-dimethyl-1-triazeno)-imidazole-4-carboxamide (DIC) in mammalian cells, revealing a greater inhibitory effect in light and identifying pathways of DIC degradation (Saunders & Chao, 1974).
Safety and Hazards
作用機序
Target of Action
The primary target of 5-Aminooxazole-4-carboxamide, also known as AICA ribonucleotide (AICAR), is the AMP-dependent protein kinase (AMPK) . AMPK is a key regulator of cellular energy homeostasis and plays a crucial role in the body’s response to metabolic stress .
Mode of Action
AICAR is an intermediate in the generation of inosine monophosphate and an analog of adenosine monophosphate (AMP). It interacts with its target, AMPK, by stimulating its activity . This interaction leads to various changes in the cell, including the regulation of glucose and lipid metabolism, which are critical for maintaining energy balance .
Biochemical Pathways
AICAR affects several biochemical pathways. It is an intermediate in the purine de novo synthesis pathway, which is responsible for the generation of inosine monophosphate . It also impacts the AMPK pathway, leading to the regulation of energy homeostasis and metabolic stress . The activation of AMPK can inhibit biosynthetic pathways and stimulate energy-generating pathways .
Pharmacokinetics
The pharmacokinetics of AICAR and its metabolites have been studied in patients with malignant melanoma or sarcoma . The plasma disappearance of AICAR was found to be biphasic, with a terminal half-life of 41.4 minutes . The total clearance was 15.4 ml/kg·min, indicating that about 50% of AICAR was eliminated by extrarenal mechanisms . The renal clearance of AICAR was 5.2–10.9 ml/kg·min .
Result of Action
The activation of AMPK by AICAR has several molecular and cellular effects. It has been used clinically to treat and protect against cardiac ischemic injury . It is also of interest as a potential treatment for diabetes by increasing the metabolic activity of tissues and changing the physical composition of muscle . Furthermore, AICAR has shown antiproliferative effects, specifically inducing apoptosis of aneuploid cells .
生化学分析
Biochemical Properties
5-Aminooxazole-4-carboxamide plays a significant role in biochemical reactions, particularly in the context of nucleotide metabolism. It interacts with various enzymes and proteins, influencing their activity and function. One of the key enzymes it interacts with is adenosine kinase, which phosphorylates this compound to form its active ribotide form. This interaction is crucial for the compound’s role in nucleotide synthesis and energy metabolism .
Cellular Effects
This compound has notable effects on different types of cells and cellular processes. It influences cell function by modulating cell signaling pathways, gene expression, and cellular metabolism. For instance, it has been shown to activate AMP-activated protein kinase (AMPK), which plays a central role in regulating energy homeostasis and metabolic stress. This activation leads to increased glucose transport and lipid metabolism in skeletal muscle cells .
Molecular Mechanism
The molecular mechanism of this compound involves its conversion to an active ribotide form by adenosine kinase. This active form mimics AMP and activates AMP-activated protein kinase (AMPK). The activation of AMPK leads to the inhibition of biosynthetic pathways and the stimulation of energy-generating pathways. Additionally, this compound has been shown to inhibit the activity of UMP synthetase, leading to pyrimidine starvation and apoptosis in certain cell types .
Temporal Effects in Laboratory Settings
In laboratory settings, the effects of this compound change over time. The compound is relatively stable, but its activity can be influenced by factors such as temperature and pH. Long-term studies have shown that this compound can induce differentiation in certain cell types, such as acute myeloid leukemia blasts, over extended periods .
Dosage Effects in Animal Models
The effects of this compound vary with different dosages in animal models. At low doses, it can activate AMPK and improve metabolic parameters without causing significant adverse effects. At high doses, it may lead to toxic effects, including liver damage and metabolic disturbances. These dosage-dependent effects highlight the importance of careful dose optimization in therapeutic applications .
Metabolic Pathways
This compound is involved in several metabolic pathways, including purine and pyrimidine metabolism. It is an intermediate in the de novo synthesis of inosine monophosphate and plays a role in regulating the balance between purine and pyrimidine nucleotides. The compound’s interaction with enzymes such as adenosine kinase and UMP synthetase is crucial for its metabolic functions .
Transport and Distribution
Within cells and tissues, this compound is transported and distributed through specific transporters and binding proteins. It is taken up by cells via adenosine transporters and is distributed to various cellular compartments. The compound’s localization and accumulation can be influenced by factors such as cell type and metabolic state .
Subcellular Localization
This compound is localized in different subcellular compartments, including the cytoplasm and mitochondria. Its activity and function can be affected by its subcellular localization, which is regulated by targeting signals and post-translational modifications. The compound’s presence in specific organelles is essential for its role in energy metabolism and nucleotide synthesis .
特性
IUPAC Name |
5-amino-1,3-oxazole-4-carboxamide | |
---|---|---|
Source | PubChem | |
URL | https://pubchem.ncbi.nlm.nih.gov | |
Description | Data deposited in or computed by PubChem | |
InChI |
InChI=1S/C4H5N3O2/c5-3(8)2-4(6)9-1-7-2/h1H,6H2,(H2,5,8) | |
Source | PubChem | |
URL | https://pubchem.ncbi.nlm.nih.gov | |
Description | Data deposited in or computed by PubChem | |
InChI Key |
YFPOLIPFJALTDR-UHFFFAOYSA-N | |
Source | PubChem | |
URL | https://pubchem.ncbi.nlm.nih.gov | |
Description | Data deposited in or computed by PubChem | |
Canonical SMILES |
C1=NC(=C(O1)N)C(=O)N | |
Source | PubChem | |
URL | https://pubchem.ncbi.nlm.nih.gov | |
Description | Data deposited in or computed by PubChem | |
Molecular Formula |
C4H5N3O2 | |
Source | PubChem | |
URL | https://pubchem.ncbi.nlm.nih.gov | |
Description | Data deposited in or computed by PubChem | |
DSSTOX Substance ID |
DTXSID00660007 | |
Record name | 5-Amino-1,3-oxazole-4-carboxamide | |
Source | EPA DSSTox | |
URL | https://comptox.epa.gov/dashboard/DTXSID00660007 | |
Description | DSSTox provides a high quality public chemistry resource for supporting improved predictive toxicology. | |
Molecular Weight |
127.10 g/mol | |
Source | PubChem | |
URL | https://pubchem.ncbi.nlm.nih.gov | |
Description | Data deposited in or computed by PubChem | |
CAS No. |
30380-27-9 | |
Record name | 5-Amino-1,3-oxazole-4-carboxamide | |
Source | EPA DSSTox | |
URL | https://comptox.epa.gov/dashboard/DTXSID00660007 | |
Description | DSSTox provides a high quality public chemistry resource for supporting improved predictive toxicology. | |
Retrosynthesis Analysis
AI-Powered Synthesis Planning: Our tool employs the Template_relevance Pistachio, Template_relevance Bkms_metabolic, Template_relevance Pistachio_ringbreaker, Template_relevance Reaxys, Template_relevance Reaxys_biocatalysis model, leveraging a vast database of chemical reactions to predict feasible synthetic routes.
One-Step Synthesis Focus: Specifically designed for one-step synthesis, it provides concise and direct routes for your target compounds, streamlining the synthesis process.
Accurate Predictions: Utilizing the extensive PISTACHIO, BKMS_METABOLIC, PISTACHIO_RINGBREAKER, REAXYS, REAXYS_BIOCATALYSIS database, our tool offers high-accuracy predictions, reflecting the latest in chemical research and data.
Strategy Settings
Precursor scoring | Relevance Heuristic |
---|---|
Min. plausibility | 0.01 |
Model | Template_relevance |
Template Set | Pistachio/Bkms_metabolic/Pistachio_ringbreaker/Reaxys/Reaxys_biocatalysis |
Top-N result to add to graph | 6 |
Feasible Synthetic Routes
Q1: What is the significance of the reaction conditions in synthesizing 5-acetamidooxazole-4-carboxamides from 2-acylamino-2-cyanoacetamides?
A1: The research article highlights the importance of specific reaction conditions in successfully synthesizing 5-acetamidooxazole-4-carboxamides []. Heating 2-acylamino-2-cyanoacetamides with acetic anhydride in the presence of perchloric acid is crucial for driving the reaction towards the desired product. This suggests that the acidic environment and the presence of the anhydride are essential for the cyclization and formation of the oxazole ring.
試験管内研究製品の免責事項と情報
BenchChemで提示されるすべての記事および製品情報は、情報提供を目的としています。BenchChemで購入可能な製品は、生体外研究のために特別に設計されています。生体外研究は、ラテン語の "in glass" に由来し、生物体の外で行われる実験を指します。これらの製品は医薬品または薬として分類されておらず、FDAから任何の医療状態、病気、または疾患の予防、治療、または治癒のために承認されていません。これらの製品を人間または動物に体内に導入する形態は、法律により厳格に禁止されています。これらのガイドラインに従うことは、研究と実験において法的および倫理的な基準の遵守を確実にするために重要です。