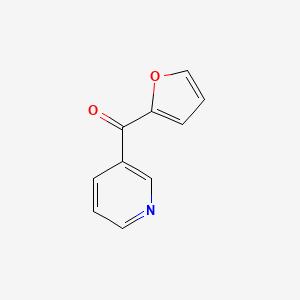
3-(2-Furanoyl)pyridine
説明
3-(2-Furanoyl)pyridine is a compound that belongs to the class of organic compounds known as furo[2,3-b]pyridines. These compounds are characterized by a fused ring structure that incorporates both a furan and a pyridine ring. The specific structure of this compound is not directly discussed in the provided papers, but the papers do provide insights into the synthesis, molecular structure, chemical reactions, and physical and chemical properties of closely related furo[2,3-b]pyridine derivatives.
Synthesis Analysis
The synthesis of furo[2,3-b]pyridines can be achieved through various methods. One approach involves the heterocyclization of pyridine-N-oxide derivatives under mild, metal-free conditions to produce a range of substituted furo[2,3-b]pyridines with different functional groups at the 2- and 3-positions . Another method described is the one-pot Sonogashira coupling/heteroannulation sequence, which allows for the efficient and rapid synthesis of furo[3,2-b]pyridine and its derivatives . Additionally, the synthesis of furo[2,3-b]pyridines can be accomplished through a three-step sequence starting from readily available materials, yielding compounds with various substituents .
Molecular Structure Analysis
The molecular structure of furo[2,3-b]pyridine derivatives has been investigated using single crystal X-ray diffraction data. For instance, the crystal and molecular structure of a related compound, 6-bromo-2-(furan-2-yl)-3-(prop-2-ynyl)-3H-imidazo[4,5-b]pyridine, was studied to understand its geometry in the solid state and the intermolecular interactions present in the crystal packing .
Chemical Reactions Analysis
Furo[2,3-b]pyridines exhibit a variety of chemical reactivities. The pyridine moiety can undergo C-H amination and borylation reactions, although some reactions like C-H fluorination and radical C-H arylation are less efficient . The furan moiety can participate in ring-opening reactions under certain conditions, such as with hydrazine, to generate new scaffolds . Intramolecular reactions, such as Diels-Alder reactions, have also been used to synthesize dihydrofuro[2,3-b]pyridines, which can be further dehydrogenated to furo[2,3-b]pyridines .
Physical and Chemical Properties Analysis
The physical and chemical properties of furo[2,3-b]pyridines depend on the substituents present on the core structure. The papers describe various substituted furo[2,3-b]pyridines with different functional groups, such as ester, amide, and ketone, which can influence properties like solubility, melting point, and reactivity . The crystal structure analysis provides insights into the density and molecular geometry, which are important for understanding the material properties of these compounds .
Safety and Hazards
作用機序
Target of Action
It’s worth noting that pyridine derivatives, including pyrido[2,3-d]pyrimidines, have been studied extensively for their broad spectrum of activities, including antitumor, antibacterial, cns depressive, anticonvulsant, and antipyretic activities . They have been found to target various proteins, including tyrosine kinase, extracellular regulated protein kinases – ABL kinase, phosphatidylinositol-3 kinase, mammalian target of rapamycin, p38 mitogen-activated protein kinases, BCR-ABL, dihydrofolate reductase, cyclin-dependent kinase, phosphodiesterase, KRAS and fibroblast growth factor receptors .
Mode of Action
Pyrimidine-based anti-inflammatory agents, which include some pyridine derivatives, generally function by suppressing the activity of cox-1 and cox-2 enzymes, thus reducing the generation of prostaglandin e2 .
Biochemical Pathways
Pyridine derivatives have been found to affect various signaling pathways, including those involving the above-mentioned targets . For instance, 2,4-diaminopyrido[2,3-d]pyrimidine has been found to induce two separate signaling pathways, leading to G2/M cell cycle arrest and induction of apoptosis .
Result of Action
Pyridine derivatives have been found to exhibit a range of pharmacological effects, including antitumor, antibacterial, cns depressive, anticonvulsant, and antipyretic activities .
Action Environment
It’s worth noting that the synthesis of pyridine derivatives from bio-based feedstocks like furfural has been reported, suggesting that environmental factors could potentially influence the production and availability of these compounds .
生化学分析
Biochemical Properties
3-(2-Furanoyl)pyridine plays a significant role in various biochemical reactions. It interacts with several enzymes, proteins, and other biomolecules, influencing their activity and function. For instance, this compound has been shown to interact with enzymes involved in oxidative stress responses, such as superoxide dismutase and catalase. These interactions can modulate the activity of these enzymes, thereby affecting the cellular redox state. Additionally, this compound can bind to certain proteins, altering their conformation and function, which may have implications for signal transduction pathways and cellular homeostasis .
Cellular Effects
This compound exerts various effects on different cell types and cellular processes. In cancer cells, it has been observed to inhibit cell proliferation and induce apoptosis. This compound can influence cell signaling pathways, such as the MAPK and PI3K/Akt pathways, leading to changes in gene expression and cellular metabolism. For example, this compound can downregulate the expression of anti-apoptotic genes and upregulate pro-apoptotic genes, promoting programmed cell death. Furthermore, it can affect cellular metabolism by inhibiting key metabolic enzymes, thereby reducing the availability of essential metabolites for cell growth and survival .
Molecular Mechanism
The molecular mechanism of action of this compound involves several key interactions at the molecular level. This compound can bind to specific biomolecules, such as DNA and proteins, through hydrogen bonding and hydrophobic interactions. By binding to DNA, this compound can interfere with DNA replication and transcription, leading to cell cycle arrest and apoptosis. Additionally, it can inhibit the activity of certain enzymes, such as kinases and proteases, by binding to their active sites and preventing substrate binding. These interactions can result in the modulation of various cellular processes, including cell division, signal transduction, and gene expression .
Temporal Effects in Laboratory Settings
In laboratory settings, the effects of this compound can change over time due to its stability and degradation. This compound is relatively stable under physiological conditions, but it can undergo degradation in the presence of certain enzymes or reactive oxygen species. Long-term exposure to this compound has been shown to have sustained effects on cellular function, including prolonged inhibition of cell proliferation and induction of apoptosis. These temporal effects are important for understanding the potential therapeutic applications of this compound and for designing appropriate dosing regimens in experimental studies .
Dosage Effects in Animal Models
The effects of this compound vary with different dosages in animal models. At low doses, this compound can exhibit beneficial effects, such as anti-inflammatory and antioxidant activities. At high doses, it can cause toxic or adverse effects, including hepatotoxicity and nephrotoxicity. Threshold effects have been observed, where a certain dosage is required to achieve the desired therapeutic effect without causing significant toxicity. These findings highlight the importance of dose optimization in preclinical studies to ensure the safety and efficacy of this compound .
Metabolic Pathways
This compound is involved in several metabolic pathways, interacting with various enzymes and cofactors. It can be metabolized by cytochrome P450 enzymes in the liver, leading to the formation of reactive metabolites. These metabolites can further interact with cellular macromolecules, such as proteins and DNA, potentially causing cellular damage. Additionally, this compound can affect metabolic flux by inhibiting key enzymes in glycolysis and the tricarboxylic acid cycle, thereby altering the levels of important metabolites and energy production .
Transport and Distribution
The transport and distribution of this compound within cells and tissues are mediated by specific transporters and binding proteins. This compound can be taken up by cells through passive diffusion or active transport mechanisms. Once inside the cell, it can bind to intracellular proteins, influencing its localization and accumulation. For example, this compound can bind to cytoplasmic proteins, leading to its retention in the cytoplasm, or it can be transported to the nucleus, where it can interact with nuclear proteins and DNA .
Subcellular Localization
The subcellular localization of this compound is crucial for its activity and function. This compound can be localized to specific cellular compartments, such as the cytoplasm, nucleus, or mitochondria, depending on its interactions with targeting signals or post-translational modifications. For instance, this compound can be directed to the mitochondria through specific targeting sequences, where it can affect mitochondrial function and induce apoptosis. Understanding the subcellular localization of this compound is essential for elucidating its mechanism of action and potential therapeutic applications .
特性
IUPAC Name |
furan-2-yl(pyridin-3-yl)methanone | |
---|---|---|
Source | PubChem | |
URL | https://pubchem.ncbi.nlm.nih.gov | |
Description | Data deposited in or computed by PubChem | |
InChI |
InChI=1S/C10H7NO2/c12-10(9-4-2-6-13-9)8-3-1-5-11-7-8/h1-7H | |
Source | PubChem | |
URL | https://pubchem.ncbi.nlm.nih.gov | |
Description | Data deposited in or computed by PubChem | |
InChI Key |
XLGFQKIWXWMNGG-UHFFFAOYSA-N | |
Source | PubChem | |
URL | https://pubchem.ncbi.nlm.nih.gov | |
Description | Data deposited in or computed by PubChem | |
Canonical SMILES |
C1=CC(=CN=C1)C(=O)C2=CC=CO2 | |
Source | PubChem | |
URL | https://pubchem.ncbi.nlm.nih.gov | |
Description | Data deposited in or computed by PubChem | |
Molecular Formula |
C10H7NO2 | |
Source | PubChem | |
URL | https://pubchem.ncbi.nlm.nih.gov | |
Description | Data deposited in or computed by PubChem | |
DSSTOX Substance ID |
DTXSID70374700 | |
Record name | 3-(2-Furanoyl)pyridine | |
Source | EPA DSSTox | |
URL | https://comptox.epa.gov/dashboard/DTXSID70374700 | |
Description | DSSTox provides a high quality public chemistry resource for supporting improved predictive toxicology. | |
Molecular Weight |
173.17 g/mol | |
Source | PubChem | |
URL | https://pubchem.ncbi.nlm.nih.gov | |
Description | Data deposited in or computed by PubChem | |
CAS RN |
72770-55-9 | |
Record name | 3-(2-Furanoyl)pyridine | |
Source | EPA DSSTox | |
URL | https://comptox.epa.gov/dashboard/DTXSID70374700 | |
Description | DSSTox provides a high quality public chemistry resource for supporting improved predictive toxicology. | |
試験管内研究製品の免責事項と情報
BenchChemで提示されるすべての記事および製品情報は、情報提供を目的としています。BenchChemで購入可能な製品は、生体外研究のために特別に設計されています。生体外研究は、ラテン語の "in glass" に由来し、生物体の外で行われる実験を指します。これらの製品は医薬品または薬として分類されておらず、FDAから任何の医療状態、病気、または疾患の予防、治療、または治癒のために承認されていません。これらの製品を人間または動物に体内に導入する形態は、法律により厳格に禁止されています。これらのガイドラインに従うことは、研究と実験において法的および倫理的な基準の遵守を確実にするために重要です。