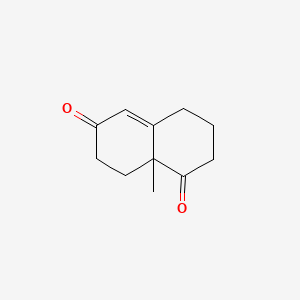
Cétone de Wieland-Miescher
Vue d'ensemble
Description
The Wieland-Miescher ketone (WMK) is a naturally occurring ketone that has been studied extensively for its potential as a therapeutic agent. It was first isolated from human urine in 1915 by German chemists Emil Wieland and Paul Miescher, and has since been the subject of numerous scientific studies. WMK is an important component of the human body, and it is believed to play a role in several physiological processes. In addition, it has been investigated for its potential to act as an anti-inflammatory, antioxidant, and anti-cancer agent.
Applications De Recherche Scientifique
Synthèse des stéroïdes et des terpénoïdes
La Cétone de Wieland-Miescher (WMK) sert d'intermédiaire crucial dans la synthèse des stéroïdes et des terpénoïdes . Ces classes de composés ont une large gamme d'activités biologiques et sont utilisées dans le développement de médicaments et d'autres agents thérapeutiques. La structure de la cétone permet la construction de cadres moléculaires complexes typiques de ces produits naturels.
Réactions catalysées par des enzymes
La WMK peut être synthétisée par des réactions catalysées par des enzymes, telles que l'annulation de Robinson, en utilisant des lipases . Cette méthode est importante pour la chimie verte, offrant une alternative écologiquement plus viable aux synthèses chimiques traditionnelles. Des enzymes comme la lipase du pancréas porcin ont montré qu'elles catalysaient efficacement cette réaction.
Criblage de biocatalyseurs
Le composé est utilisé dans le criblage de biocatalyseurs pour des applications synthétiques . En testant la capacité de diverses enzymes à synthétiser la WMK, les chercheurs peuvent identifier de nouveaux biocatalyseurs pouvant être utilisés dans la fabrication pharmaceutique, conduisant à des méthodes de production plus durables.
Synthèse pharmaceutique
En recherche pharmaceutique, la WMK est utilisée dans la synthèse d'ingrédients pharmaceutiques actifs (API). Son utilisation dans les réactions de synthèse en un seul pot permet de rationaliser le processus de production, le rendant plus efficace et rentable .
Développement d'agents anticancéreux
La WMK a été utilisée dans la synthèse totale de plus de 50 produits naturels présentant des propriétés anticancéreuses prometteuses . Son incorporation dans les voies de synthèse permet la création de nouveaux composés qui pourraient servir de traitements anticancéreux potentiels.
Recherche antimicrobienne et antivirale
L'application de la cétone s'étend au développement de composés ayant des activités antimicrobiennes et antivirales . Son utilisation en chimie synthétique permet l'exploration de nouveaux médicaments qui pourraient lutter contre diverses infections et virus.
Recherche sur les maladies neurodégénératives
Des chercheurs ont utilisé la WMK dans la synthèse de composés présentant des effets antineurodégénératifs potentiels . Ceci est particulièrement pertinent pour des maladies comme Alzheimer et Parkinson, où de nouvelles options thérapeutiques sont constamment recherchées.
Activité immunomodulatrice
Enfin, la WMK est un synthon clé dans la création de molécules ayant des effets immunomodulateurs . Ces composés peuvent moduler le système immunitaire, ce qui est bénéfique pour le traitement des maladies auto-immunes et l'amélioration de l'efficacité des vaccins.
Mécanisme D'action
Target of Action
The Wieland-Miescher ketone (WMK) is a versatile synthon that has been employed in the total synthesis of more than 50 natural products . These natural products predominantly include sesquiterpenoids, diterpenes, and steroids . Therefore, the primary targets of WMK are these natural products and their associated biological pathways.
Mode of Action
WMK interacts with its targets through a process known as Robinson annulation . This process involves a Michael addition followed by an Aldol reaction . The promiscuous catalytic activity of lipase in the Robinson reaction plays a crucial role in both these steps .
Biochemical Pathways
The biochemical pathways affected by WMK are those involved in the synthesis of sesquiterpenoids, diterpenes, and steroids . The Robinson annulation process, catalyzed by lipase, leads to the formation of WMK, which then serves as a key intermediate in these synthesis pathways .
Pharmacokinetics
It’s worth noting that wmk is synthesized in organic media, suggesting that its bioavailability may be influenced by the properties of this medium .
Result of Action
The result of WMK’s action is the synthesis of a variety of biologically active compounds, including steroids, terpenoids, and taxol . These compounds possess potential biological properties, including anticancer, antimicrobial, antiviral, antineurodegenerative, and immunomodulatory activities .
Action Environment
The action of WMK is influenced by the organic media in which it is synthesized . The use of lipase from porcine pancreas (PPL) to catalyze a Robinson annulation, which forms WMK in organic media, has practical significance for environmentally friendly syntheses and the evolution of biosyntheses .
Orientations Futures
Analyse Biochimique
Biochemical Properties
Wieland-Miescher ketone plays a significant role in biochemical reactions, particularly in the synthesis of complex natural products. It interacts with various enzymes, proteins, and other biomolecules. For instance, lipase from porcine pancreas catalyzes the Robinson annulation reaction, which forms Wieland-Miescher ketone in organic media . This reaction involves the Michael addition and aldol condensation steps, highlighting the enzyme’s catalytic promiscuity. The interaction between Wieland-Miescher ketone and lipase demonstrates the compound’s potential in environmentally friendly syntheses and the evolution of biosyntheses.
Cellular Effects
Wieland-Miescher ketone influences various cellular processes and functions. It has been employed in the synthesis of biologically active compounds, such as steroids and terpenoids, which exhibit anticancer, antimicrobial, antiviral, antineurodegenerative, and immunomodulatory activities . These compounds can affect cell signaling pathways, gene expression, and cellular metabolism. For example, steroids synthesized from Wieland-Miescher ketone can modulate gene expression by binding to specific receptors and influencing transcriptional activity.
Molecular Mechanism
The molecular mechanism of Wieland-Miescher ketone involves its participation in the Robinson annulation reaction. This reaction starts with a Michael addition, followed by an intramolecular aldol condensation, resulting in the formation of a new six-membered ring containing an alpha, beta-unsaturated ketone . The reaction is catalyzed by enzymes such as lipase, which facilitates the formation of Wieland-Miescher ketone through its catalytic triad. The interaction between the carbonyl group and the oxyanion hole increases the electrophilic ability of methyl vinyl ketone, leading to the formation of the final product.
Temporal Effects in Laboratory Settings
In laboratory settings, the effects of Wieland-Miescher ketone can change over time. The compound’s stability and degradation are crucial factors in its long-term effects on cellular function. Studies have shown that Wieland-Miescher ketone is stable under specific conditions, such as ambient temperature in anhydrous dimethylformamide solvent . Its stability may vary depending on the experimental conditions, such as solvent type and temperature. Long-term effects on cellular function can be observed in in vitro and in vivo studies, where the compound’s stability and degradation play a significant role.
Dosage Effects in Animal Models
The effects of Wieland-Miescher ketone vary with different dosages in animal models. Studies have shown that the compound exhibits threshold effects, where low doses may have minimal impact, while higher doses can lead to significant biological effects . For instance, high doses of Wieland-Miescher ketone-derived steroids may exhibit toxic or adverse effects, such as hormonal imbalances or organ toxicity. Therefore, it is essential to determine the optimal dosage to achieve the desired biological effects while minimizing potential adverse effects.
Metabolic Pathways
Wieland-Miescher ketone is involved in various metabolic pathways, particularly in the synthesis of natural products. The compound interacts with enzymes and cofactors, such as lipase and L-proline, which facilitate its conversion into biologically active compounds . These metabolic pathways can influence metabolic flux and metabolite levels, leading to the formation of complex natural products with diverse biological activities.
Transport and Distribution
The transport and distribution of Wieland-Miescher ketone within cells and tissues are crucial for its biological activity. The compound interacts with transporters and binding proteins that facilitate its localization and accumulation in specific cellular compartments . These interactions can influence the compound’s bioavailability and efficacy in various biological processes.
Subcellular Localization
Wieland-Miescher ketone’s subcellular localization plays a significant role in its activity and function. The compound may be directed to specific compartments or organelles through targeting signals or post-translational modifications . These localization mechanisms can affect the compound’s interaction with biomolecules and its overall biological activity.
Propriétés
IUPAC Name |
8a-methyl-3,4,7,8-tetrahydro-2H-naphthalene-1,6-dione | |
---|---|---|
Source | PubChem | |
URL | https://pubchem.ncbi.nlm.nih.gov | |
Description | Data deposited in or computed by PubChem | |
InChI |
InChI=1S/C11H14O2/c1-11-6-5-9(12)7-8(11)3-2-4-10(11)13/h7H,2-6H2,1H3 | |
Source | PubChem | |
URL | https://pubchem.ncbi.nlm.nih.gov | |
Description | Data deposited in or computed by PubChem | |
InChI Key |
DNHDRUMZDHWHKG-UHFFFAOYSA-N | |
Source | PubChem | |
URL | https://pubchem.ncbi.nlm.nih.gov | |
Description | Data deposited in or computed by PubChem | |
Canonical SMILES |
CC12CCC(=O)C=C1CCCC2=O | |
Source | PubChem | |
URL | https://pubchem.ncbi.nlm.nih.gov | |
Description | Data deposited in or computed by PubChem | |
Molecular Formula |
C11H14O2 | |
Source | PubChem | |
URL | https://pubchem.ncbi.nlm.nih.gov | |
Description | Data deposited in or computed by PubChem | |
DSSTOX Substance ID |
DTXSID40894783 | |
Record name | 3,4,8,8a-Tetrahydro-8a-methyl-(2H,7H)naphthalene-1,6-dione | |
Source | EPA DSSTox | |
URL | https://comptox.epa.gov/dashboard/DTXSID40894783 | |
Description | DSSTox provides a high quality public chemistry resource for supporting improved predictive toxicology. | |
Molecular Weight |
178.23 g/mol | |
Source | PubChem | |
URL | https://pubchem.ncbi.nlm.nih.gov | |
Description | Data deposited in or computed by PubChem | |
CAS RN |
20007-72-1, 33878-99-8 | |
Record name | (±)-Wieland-Miescher ketone | |
Source | CAS Common Chemistry | |
URL | https://commonchemistry.cas.org/detail?cas_rn=20007-72-1 | |
Description | CAS Common Chemistry is an open community resource for accessing chemical information. Nearly 500,000 chemical substances from CAS REGISTRY cover areas of community interest, including common and frequently regulated chemicals, and those relevant to high school and undergraduate chemistry classes. This chemical information, curated by our expert scientists, is provided in alignment with our mission as a division of the American Chemical Society. | |
Explanation | The data from CAS Common Chemistry is provided under a CC-BY-NC 4.0 license, unless otherwise stated. | |
Record name | 9-Methyl-delta5,10-octalin-1,6-dione | |
Source | ChemIDplus | |
URL | https://pubchem.ncbi.nlm.nih.gov/substance/?source=chemidplus&sourceid=0020007721 | |
Description | ChemIDplus is a free, web search system that provides access to the structure and nomenclature authority files used for the identification of chemical substances cited in National Library of Medicine (NLM) databases, including the TOXNET system. | |
Record name | 9(S)-Methyl-delta-5(10)-octalin-1,6-dione | |
Source | ChemIDplus | |
URL | https://pubchem.ncbi.nlm.nih.gov/substance/?source=chemidplus&sourceid=0033878998 | |
Description | ChemIDplus is a free, web search system that provides access to the structure and nomenclature authority files used for the identification of chemical substances cited in National Library of Medicine (NLM) databases, including the TOXNET system. | |
Record name | 20007-72-1 | |
Source | DTP/NCI | |
URL | https://dtp.cancer.gov/dtpstandard/servlet/dwindex?searchtype=NSC&outputformat=html&searchlist=87581 | |
Description | The NCI Development Therapeutics Program (DTP) provides services and resources to the academic and private-sector research communities worldwide to facilitate the discovery and development of new cancer therapeutic agents. | |
Explanation | Unless otherwise indicated, all text within NCI products is free of copyright and may be reused without our permission. Credit the National Cancer Institute as the source. | |
Record name | 3,4,8,8a-Tetrahydro-8a-methyl-(2H,7H)naphthalene-1,6-dione | |
Source | EPA DSSTox | |
URL | https://comptox.epa.gov/dashboard/DTXSID40894783 | |
Description | DSSTox provides a high quality public chemistry resource for supporting improved predictive toxicology. | |
Record name | 3,4,8,8a-tetrahydro-8a-methyl-(2H,7H)naphthalene-1,6-dione | |
Source | European Chemicals Agency (ECHA) | |
URL | https://echa.europa.eu/substance-information/-/substanceinfo/100.039.497 | |
Description | The European Chemicals Agency (ECHA) is an agency of the European Union which is the driving force among regulatory authorities in implementing the EU's groundbreaking chemicals legislation for the benefit of human health and the environment as well as for innovation and competitiveness. | |
Explanation | Use of the information, documents and data from the ECHA website is subject to the terms and conditions of this Legal Notice, and subject to other binding limitations provided for under applicable law, the information, documents and data made available on the ECHA website may be reproduced, distributed and/or used, totally or in part, for non-commercial purposes provided that ECHA is acknowledged as the source: "Source: European Chemicals Agency, http://echa.europa.eu/". Such acknowledgement must be included in each copy of the material. ECHA permits and encourages organisations and individuals to create links to the ECHA website under the following cumulative conditions: Links can only be made to webpages that provide a link to the Legal Notice page. | |
Record name | 9-METHYL-.DELTA.5,10-OCTALIN-1,6-DIONE | |
Source | FDA Global Substance Registration System (GSRS) | |
URL | https://gsrs.ncats.nih.gov/ginas/app/beta/substances/FAX7VUD577 | |
Description | The FDA Global Substance Registration System (GSRS) enables the efficient and accurate exchange of information on what substances are in regulated products. Instead of relying on names, which vary across regulatory domains, countries, and regions, the GSRS knowledge base makes it possible for substances to be defined by standardized, scientific descriptions. | |
Explanation | Unless otherwise noted, the contents of the FDA website (www.fda.gov), both text and graphics, are not copyrighted. They are in the public domain and may be republished, reprinted and otherwise used freely by anyone without the need to obtain permission from FDA. Credit to the U.S. Food and Drug Administration as the source is appreciated but not required. | |
Retrosynthesis Analysis
AI-Powered Synthesis Planning: Our tool employs the Template_relevance Pistachio, Template_relevance Bkms_metabolic, Template_relevance Pistachio_ringbreaker, Template_relevance Reaxys, Template_relevance Reaxys_biocatalysis model, leveraging a vast database of chemical reactions to predict feasible synthetic routes.
One-Step Synthesis Focus: Specifically designed for one-step synthesis, it provides concise and direct routes for your target compounds, streamlining the synthesis process.
Accurate Predictions: Utilizing the extensive PISTACHIO, BKMS_METABOLIC, PISTACHIO_RINGBREAKER, REAXYS, REAXYS_BIOCATALYSIS database, our tool offers high-accuracy predictions, reflecting the latest in chemical research and data.
Strategy Settings
Precursor scoring | Relevance Heuristic |
---|---|
Min. plausibility | 0.01 |
Model | Template_relevance |
Template Set | Pistachio/Bkms_metabolic/Pistachio_ringbreaker/Reaxys/Reaxys_biocatalysis |
Top-N result to add to graph | 6 |
Feasible Synthetic Routes
Q & A
Q1: What is the Wieland-Miescher ketone and how is it synthesized?
A1: The Wieland-Miescher ketone is a bicyclic diketone, specifically 9-methyl-Δ5(10)-octalin-1,6-dione, existing as a racemic mixture of (S)- and (R)-enantiomers at the 9-position. [] The most common synthetic route to WMK is through a Robinson annulation reaction. This involves the base-catalyzed condensation of 2-methyl-1,3-cyclohexanedione with methyl vinyl ketone. []
Q2: Are there alternative methods for obtaining enantiomerically pure WMK?
A2: Yes, besides the classical resolution of racemic WMK, enantiomerically enriched WMK can be obtained through several approaches:
- Kinetic resolution using yeast: Torulaspora delbrueckii IFO 10921 selectively reduces the (S)-enantiomer of WMK, allowing for the isolation of the (R)-enantiomer in high enantiomeric excess. []
- Asymmetric Robinson Annulation: The use of chiral catalysts, such as proline derivatives, in the Robinson annulation reaction can lead to the formation of enantioenriched WMK. [, , ]
Q3: What is the absolute stereochemistry of naturally occurring (-)-Wieland-Miescher ketone?
A3: The absolute stereochemistry of naturally occurring (-)-Wieland-Miescher ketone was determined to be 8aR using X-ray crystallography of its bis(4-bromobenzoate) derivatives and further corroborated by circular dichroism (CD) spectroscopy. []
Q4: How can the structure of WMK be modified for further synthetic applications?
A4: The WMK structure offers various avenues for modification:
- Functional group transformations: The enone and isolated ketone functionalities can be selectively manipulated. For example, the enone can undergo reduction, oxidation, electrophilic additions, and nucleophilic additions. []
- C-8 functionalization: Regio- and stereoselective methods have been developed for introducing substituents, such as hydroxymethyl, hydroxyl, and thiophenyl groups, at the C-8 position of WMK derivatives. []
- C-9 quaternary center construction: The Mukaiyama aldol reaction provides an efficient way to introduce a lateral chain at the C-9 position of WMK derivatives, creating a quaternary center. []
- Protection strategies: Protecting groups can be employed to mask the reactivity of the ketone groups. For instance, the non-conjugated ketone can be protected as a ketal with glycol, while the conjugated ketone can be protected as a thioketal with ethanedithiol. []
Q5: Why is the introduction of substituents at the C-8 position of WMK significant?
A5: Functionalization at the C-8 position is crucial as it allows for the controlled introduction of stereochemical diversity, a key aspect in synthesizing natural products with specific biological activities. []
Q6: What makes WMK a valuable starting material in total synthesis?
A6: WMK's rigid bicyclic framework and the presence of multiple reactive sites make it an ideal starting point for constructing complex polycyclic structures found in numerous natural products. [, , , ]
Q7: What are some notable examples of natural products synthesized using WMK as a starting material?
A7: WMK has served as a key building block in the total synthesis of diverse natural products, including:
- Terpenes: Manool, a labdane diterpene with platelet aggregation inhibitory activity. []
- Sesquiterpenes: Bakkenolide A, a compound with antitumor and antiviral properties. []
- Meroterpenoids: Dysideanone B and Dysiherbol A, marine natural products exhibiting anti-cancer activity. []
- Alkaloids: Aristomakine, an alkaloid with potential medicinal properties. []
- Other compounds: Pallavicinin and Neopallavicinin (diterpenoids), [] Subglutinols A and B (diterpene pyrones), [] Stachyflin (potential anti-influenza agent), [] and many others. [, , , , , , , , , ]
Q8: Can you provide a specific example of how WMK was utilized in a total synthesis?
A8: In the total synthesis of (-)-scabronine G, a compound known to induce neurotrophic factor production, the researchers utilized (-)-Wieland-Miescher ketone as the starting point. The synthesis involved a series of transformations, including alkylation, reduction, and ring-closing metathesis, showcasing the versatility of WMK in building complex structures. []
Q9: Can WMK or its derivatives act as catalysts in organic reactions?
A9: While WMK itself is not typically used as a catalyst, its derivatives, particularly those containing amino groups, can function as organocatalysts. For example, monoacylated derivatives of 1,2-cyclohexanediamine derived from WMK exhibit catalytic activity in intramolecular aldol condensations, including the synthesis of WMK itself. []
Q10: Are there any "green chemistry" aspects associated with WMK synthesis or its applications?
A10: Yes, the use of organocatalysts derived from WMK aligns with green chemistry principles by reducing reliance on potentially toxic metal catalysts. Additionally, research has explored the use of ionic liquids as recyclable solvents in asymmetric cyclization reactions leading to WMK analogues, further contributing to greener synthetic methodologies. []
Q11: Has computational chemistry played a role in understanding WMK and its derivatives?
A11: Yes, computational methods like Density Functional Theory (DFT) calculations have been employed to:* Elucidate the mechanism of reactions involving WMK derivatives, such as the lead tetraacetate mediated oxidative cleavage of unsaturated diols derived from WMK. []* Rationalize the stereochemical outcomes of reactions. For example, in the synthesis of (+)-stachyflin, DFT calculations helped explain the BF3·Et2O-induced cascade reaction used to build the pentacyclic ring system. []
Avertissement et informations sur les produits de recherche in vitro
Veuillez noter que tous les articles et informations sur les produits présentés sur BenchChem sont destinés uniquement à des fins informatives. Les produits disponibles à l'achat sur BenchChem sont spécifiquement conçus pour des études in vitro, qui sont réalisées en dehors des organismes vivants. Les études in vitro, dérivées du terme latin "in verre", impliquent des expériences réalisées dans des environnements de laboratoire contrôlés à l'aide de cellules ou de tissus. Il est important de noter que ces produits ne sont pas classés comme médicaments et n'ont pas reçu l'approbation de la FDA pour la prévention, le traitement ou la guérison de toute condition médicale, affection ou maladie. Nous devons souligner que toute forme d'introduction corporelle de ces produits chez les humains ou les animaux est strictement interdite par la loi. Il est essentiel de respecter ces directives pour assurer la conformité aux normes légales et éthiques en matière de recherche et d'expérimentation.