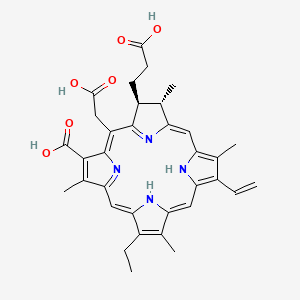
Chlorin e6
Descripción general
Descripción
Chlorin e6 (Ce6) is a photosensitizer with strong absorption peaks at wavelengths of 402 and 662 nm. It also exhibits intense fluorescence at 668 nm . Ce6 has antimicrobial efficacy and anticancer activity . It induces cell apoptosis via caspase-3 activation and can be used for cancer research .
Synthesis Analysis
This compound can be synthesized from Spirulina platensis . Two efficient synthetic methodologies have been described for the preparation of Ce6 . Another green method involves synthesizing Ce6 from chlorophyll a, which was extracted from crude silkworm excrement using concentrated (strong) alkali hydrolysis and acidification .Molecular Structure Analysis
The study of optical and photophysical properties of Ce6 has been conducted, taking into account the dynamical and vibrational effects both by using Wigner distribution or coupling with molecular dynamics .Physical and Chemical Properties Analysis
Ce6 has strong absorption peaks at wavelengths of 402 and 662 nm, as well as exhibiting intense fluorescence at 668 nm . The linear and non-linear optical properties have been studied .Aplicaciones Científicas De Investigación
Fluorescence-based Quantification in Liposomes
Chlorin e6's application in fluorescence-based methods for sensitive and rapid estimation in stealth liposomes highlights its significance in cancer therapy, especially for neck, head, early-stage lung, and skin cancers. The study developed a precise method for quantifying this compound in liposomal formulations, underlining its strong fluorescence properties, which facilitate efficient encapsulation efficiency estimation in stealth liposomes (Jain et al., 2020).
Antitumor and Other Biomedical Applications
This compound's role as an effective photosensitizer and sonosensitizer in antitumor therapy through photodynamic therapy, dynamic acoustic therapy, and combined acoustic and light therapy for tumors is well documented. Beyond tumor therapy, this compound's applications extend to fluorescence navigation, antibacterials, and plant growth regulation, showcasing its versatility across biomedical fields (Liao et al., 2023).
Nanoparticle Conjugation for Enhanced Therapy
The conjugation of this compound to gold nanostars for simultaneous photodynamic and plasmonic photothermal therapy (PDT/PPTT) upon single laser irradiation exemplifies nanoengineering's role in enhancing anticancer efficiency. This conjugation demonstrates the synergistic effects of early-phase PDT and late-phase PPTT, offering a novel approach to cancer treatment (Wang et al., 2013).
Impurity Analysis for Quality Control
Understanding this compound's impurities is crucial for its application in Photolon formulation, used in photodynamic therapy for various diseases. The identification and characterization of both process- and degradation-related impurities in this compound contribute to the quality control and efficacy of PDT treatments (Isakau et al., 2007).
Photophysics for Phototherapy
The study of this compound's optical and photophysical properties, including one- and two-photon absorption, fluorescence, and phosphorescence, highlights its potential as an efficient sensitizer in phototherapy. The investigation into its linear and non-linear optical properties, coupled with a detailed photophysical study, suggests its applicability in producing singlet oxygen and in two-photon absorption-based phototherapy (Gattuso et al., 2017).
Mecanismo De Acción
Target of Action
Chlorin e6 (Ce6) is a well-known photosensitizer used in photodynamic diagnosis and therapy . It primarily targets cancer cells and pathogenic bacteria . Ce6 has been shown to bind to cancer cells and bacteria, leading to their destruction upon light activation .
Mode of Action
The mode of action of Ce6 involves its interaction with light and oxygen. These reactive oxygen species (ROS) can cause damage to cellular components, including proteins, lipids, and DNA, leading to cell death .
Biochemical Pathways
The biochemical pathways affected by Ce6 involve the generation of ROS and the subsequent induction of oxidative stress . Oxidative stress can lead to DNA damage, lipid peroxidation, and protein oxidation, disrupting cellular functions and leading to cell death . Furthermore, Ce6 can prevent DNA damage repair, thus enhancing the sensitivity of tumor cells to ROS .
Pharmacokinetics
The pharmacokinetics of Ce6 involves its absorption, distribution, metabolism, and excretion (ADME). Ce6 is known to be a stable compound with a half-life of more than 3 hours . It is also known to have a high reactive oxygen species (ROS) generation ability and anticancer potency against many types of cancer . .
Result of Action
The result of Ce6’s action is the destruction of targeted cells. Upon light activation, Ce6 can induce significant cytotoxicity in cancer cells and bacteria . In cancer cells, this leads to tumor suppression . In bacteria, Ce6’s action results in bactericidal activity .
Action Environment
The action of Ce6 is influenced by environmental factors such as light and oxygen availability . The presence of light is crucial for the activation of Ce6, and its absence significantly reduces its bactericidal and cytotoxic effects . Similarly, the presence of oxygen is necessary for the generation of ROS . Furthermore, Ce6 can respond to the acidic microenvironment of bacterial infection .
Safety and Hazards
Direcciones Futuras
Análisis Bioquímico
Biochemical Properties
Ce6 interacts with various biomolecules in the cell. It has been shown to bind to cellular membranes within 30 minutes of incubation with cells . As the length of incubation increases, Ce6 gradually enters the cells and reaches saturation by 3 hours . Co-localization analysis demonstrated that Ce6 is more likely to be present in the mitochondria than in the lysosomes . This suggests that Ce6 may interact with enzymes, proteins, and other biomolecules within these organelles.
Cellular Effects
Ce6 has significant effects on various types of cells and cellular processes. For instance, Ce6-mediated photodynamic therapy (PDT) can kill cancer cells in vitro . The cytotoxicity of Ce6 is positively correlated with its concentration and light dose, indicating that the effect of Ce6 is concentration/dose-dependent . At lower concentrations and light doses, Ce6 can significantly induce apoptosis in cells, while higher doses increase necrosis or the percentage of dead cells .
Molecular Mechanism
The most common mechanism of action of Ce6 involves the activation of molecular oxygen by the photoexcited drug to produce singlet oxygen . Subsequently, the highly reactive singlet oxygen will produce a considerable oxidative stress, ultimately inducing apoptosis . From a molecular point of view, different targets of singlet oxygen have been reported including proteins, lipid membranes as well as nucleic acids .
Temporal Effects in Laboratory Settings
Over time, the effects of Ce6 can change in laboratory settings. For instance, Ce6 can combine with the cellular membrane following 30 minutes of incubation with cells . As the length of incubation increases, Ce6 gradually enters the cells in a particular distribution and reaches saturation by 3 hours . This suggests that the effects of Ce6 on cellular function can change over time, depending on factors such as the duration of incubation and the concentration of Ce6.
Dosage Effects in Animal Models
The effects of Ce6 can vary with different dosages in animal models. For instance, a study on canine tumors treated with Ce6-PDT showed that the tumors significantly diminished and the dogs’ health improved after receiving the treatment . The study also found that the cell toxicity was positively correlated with Ce6 concentration and light dose, indicating that the effect of Ce6 is concentration/dose-dependent .
Metabolic Pathways
Ce6 is involved in various metabolic pathways. For instance, it has been shown to interact with mitochondrial complex II to induce extra reactive oxygen species production . This suggests that Ce6 may interact with enzymes or cofactors within these pathways and could potentially affect metabolic flux or metabolite levels.
Transport and Distribution
Ce6 is transported and distributed within cells and tissues in a specific manner. It has been shown to bind to cellular membranes within 30 minutes of incubation with cells . As the length of incubation increases, Ce6 gradually enters the cells and reaches saturation by 3 hours . This suggests that Ce6 may interact with transporters or binding proteins within cells, and could potentially affect its localization or accumulation.
Subcellular Localization
Ce6 has a specific subcellular localization within cells. Co-localization analysis demonstrated that Ce6 is more likely to be present in the mitochondria than in the lysosomes . This suggests that Ce6 may have targeting signals or post-translational modifications that direct it to specific compartments or organelles within the cell.
Propiedades
{ "Design of the Synthesis Pathway": "The synthesis of Chlorin e6 can be achieved through a multi-step process involving several chemical reactions.", "Starting Materials": [ "Porphyrin", "2,3-dimethyl-1,4-naphthoquinone", "Methanesulfonic acid", "Sodium hydroxide", "Chloroform" ], "Reaction": [ "The first step involves the reaction of porphyrin with 2,3-dimethyl-1,4-naphthoquinone in the presence of methanesulfonic acid as a catalyst. This reaction results in the formation of a porphyrin intermediate.", "The porphyrin intermediate is then subjected to a reaction with sodium hydroxide, which leads to the formation of an anionic species.", "The anionic species is then treated with chloroform to form Chlorin e6. The final product is then purified and characterized using various analytical techniques such as NMR spectroscopy and mass spectrometry." ] } | |
Número CAS |
19660-77-6 |
Fórmula molecular |
C34H36N4O6 |
Peso molecular |
596.7 g/mol |
Nombre IUPAC |
(17S)-18-(2-carboxyethyl)-20-(carboxymethyl)-12-ethenyl-7-ethyl-3,8,13,17-tetramethyl-17,18,22,23-tetrahydroporphyrin-2-carboxylic acid |
InChI |
InChI=1S/C34H36N4O6/c1-7-19-15(3)23-12-25-17(5)21(9-10-29(39)40)32(37-25)22(11-30(41)42)33-31(34(43)44)18(6)26(38-33)14-28-20(8-2)16(4)24(36-28)13-27(19)35-23/h7,12-14,17,21,35-36H,1,8-11H2,2-6H3,(H,39,40)(H,41,42)(H,43,44)/t17-,21?/m0/s1 |
Clave InChI |
OYINILBBZAQBEV-PBVYKCSPSA-N |
SMILES isomérico |
CCC1=C(C2=CC3=C(C(=C(N3)C=C4[C@H](C(C(=N4)C(=C5C(=C(C(=N5)C=C1N2)C)C(=O)O)CC(=O)O)CCC(=O)O)C)C)C=C)C |
SMILES |
O=C(O)C/C1=C2[C@@H](CCC(O)=O)[C@H](C)C(/C=C3C(C)=C(C=C)/C(N/3)=C/C(C(C)=C/4CC)=NC4=C/C5=C(C)C(C(O)=O)=C1N5)=N\2 |
SMILES canónico |
CCC1=C(C2=CC3=C(C(=C(N3)C=C4C(C(C(=N4)C(=C5C(=C(C(=N5)C=C1N2)C)C(=O)O)CC(=O)O)CCC(=O)O)C)C)C=C)C |
Apariencia |
black solid powder |
19660-77-6 | |
Pictogramas |
Irritant |
Pureza |
>9 5 % (or refer to the Certificate of Analysis) |
Vida útil |
>2 years if stored properly |
Solubilidad |
Soluble in DMSO, not in water |
Almacenamiento |
Dry, dark and at 0 - 4 C for short term (days to weeks) or -20 C for long term (months to years). |
Sinónimos |
13-carboxy-17-(2-carboxyethyl)-15-carboxymethyl-17,18-trans-dihydro-3-vinyl-8-ethyl-2,7,12,18-tetramethylporphyrin 2-porphinepropionic acid, 18-carboxy-20-(carboxymethyl)-13-ethyl-2beta,3-dihydro-3beta,7,12,17-tetramethyl-8-vinyl- 21h,23h-porphine-2-propanoic acid, 18-carboxy-20-(carboxymethyl)-8-ethenyl-13-ethyl-2,3-dihydro-3,7,12,17-tetramethyl-, (2S-trans)- 21h,23h-porphine-7-propanoic acid, 3-carboxy-5-(carboxymethyl)-13-ethenyl-18-ethyl-7,8-dihydro-2,8,12,17-tetramethyl-, (7S,8S)- BLC 1010 BLC-1010 BLC1010 chlorin A6 chlorin e6 chlorin e6 bis-N-methylglucamine salt chlorin e6, sodium salt, (2S-trans)-isomer chlorin e6, tripotassium salt, (2S-trans)-isomer chlorin e6, trisodium salt, (2S-trans)-isomer chlorin e6-2.5 N-methyl-D-glucamine chlorin e6-polyvinylpyrrolidone chlorine A6 chlorine e6 fotolon photochlorine Photolon phytochlorin |
Origen del producto |
United States |
Retrosynthesis Analysis
AI-Powered Synthesis Planning: Our tool employs the Template_relevance Pistachio, Template_relevance Bkms_metabolic, Template_relevance Pistachio_ringbreaker, Template_relevance Reaxys, Template_relevance Reaxys_biocatalysis model, leveraging a vast database of chemical reactions to predict feasible synthetic routes.
One-Step Synthesis Focus: Specifically designed for one-step synthesis, it provides concise and direct routes for your target compounds, streamlining the synthesis process.
Accurate Predictions: Utilizing the extensive PISTACHIO, BKMS_METABOLIC, PISTACHIO_RINGBREAKER, REAXYS, REAXYS_BIOCATALYSIS database, our tool offers high-accuracy predictions, reflecting the latest in chemical research and data.
Strategy Settings
Precursor scoring | Relevance Heuristic |
---|---|
Min. plausibility | 0.01 |
Model | Template_relevance |
Template Set | Pistachio/Bkms_metabolic/Pistachio_ringbreaker/Reaxys/Reaxys_biocatalysis |
Top-N result to add to graph | 6 |
Feasible Synthetic Routes
A: Chlorin e6, upon activation by light of a specific wavelength, generates reactive oxygen species (ROS), primarily singlet oxygen (1O2). These ROS are highly cytotoxic and cause damage to various cellular components, leading to cell death [, , , , ].
A: Studies show that this compound, depending on its subcellular localization, can damage various cellular components. These include the plasma membrane [], mitochondria [], lysosomes [], and the endoplasmic reticulum []. The specific target and extent of damage can influence the mode of cell death, leading to either apoptosis or necrosis [, ].
A: Yes, pH plays a significant role in this compound distribution and activity. In acidic environments, like that of a tumor or within lysosomes, this compound exhibits increased binding to cell membranes and deeper penetration into lipid bilayers []. This pH-dependent behavior can influence its efficacy in PDT.
ANone: The molecular formula of this compound is C34H36N4O6 and its molecular weight is 612.67 g/mol.
A: this compound exhibits a characteristic absorption spectrum with a strong Soret band around 400 nm and multiple Q bands in the visible region, with the most prominent one around 660 nm [, , ]. This spectral feature allows for efficient excitation with red light, which penetrates deeper into tissues.
A: Stability studies of this compound, particularly its novel derivative DYSP-C34, show good stability in rat plasma under different storage conditions including room temperature, repeated freeze-thaw cycles, and long-term cryopreservation [].
A: While this compound itself is not known for catalytic activity, its iron complex, Fe-chlorin e6-Na (FeCNa), exhibits superoxide dismutase (SOD)-like activity. This activity is attributed to the iron element encased within the molecule and allows it to mimic SOD function [].
ANone: This aspect is not extensively covered in the provided research abstracts.
ANone: Research indicates that structural modifications significantly influence this compound's properties and PDT efficacy. For instance:
- Introduction of a 32-aryl substitution and amino acid substituent at C-152: These modifications in the novel derivative DYSP-C34 enhance cellular permeability and water solubility, making it a more promising PDT agent [].
- Conjugation with aspartic acid: Mono-L-aspartyl this compound (Talaporfin) shows altered cellular localization compared to this compound, leading to different phototoxic effects [].
- Amide and boronated amide derivatives: These derivatives exhibit higher phototoxicity against tumor cells compared to this compound, attributed to their enhanced membrane permeabilization abilities [].
- π-extension derivatives: These modifications, achieved through regioselective bromination and Suzuki–Miyaura cross-coupling reactions, result in bathochromic shifts in the absorption spectrum, improved photostability, and higher phototoxicity in vitro [].
ANone: Several formulation approaches have been investigated to address this compound's limitations and enhance its therapeutic potential:
- Liposomes: Liposomal encapsulation of this compound has been studied to improve its solubility, stability, and target specificity [, ].
- Micelles: Micellar formulations using stearic acid-grafted chitosan oligosaccharide (CSO-SA) and folate-conjugated CSO-SA (FA-CSO-SA) have shown promise in enhancing this compound delivery and photodynamic efficacy in vitro [].
- Nanosuspensions: The use of biodegradable sucrose esters as drug carriers in nanosuspension formulations has shown to improve the dissolution rate and photodynamic efficacy of this compound [].
- Quantum dots: Complexation with quantum dots (QDs), such as ZnSe/ZnS QDs, has shown to enhance this compound uptake by cancer cells and increase PDT efficacy due to efficient energy transfer between QDs and this compound [].
ANone: This aspect is not covered in the provided research abstracts.
A: Studies in mice bearing Ehrlich ascites tumors show that this compound, following intravenous injection, accumulates rapidly in various organs, with the highest concentration observed in the liver [, ]. Importantly, it exhibits a longer retention time in tumor tissue compared to other organs, highlighting its potential for targeted therapy.
A: Pharmacokinetic analysis of DYSP-C34 in rats after intravenous administration reveals a plasma half-life (t1/2z) of 6.98 h, suggesting a moderate elimination rate []. Tissue distribution studies in tumor-bearing mice show significant accumulation in tumor tissues, with higher concentrations observed in the liver and kidneys, and lower concentrations in the heart, spleen, and lungs.
ANone: Numerous studies have demonstrated the in vitro phototoxic effects of this compound and its derivatives against various cancer cell lines, including:
- Human lung cancer cells (A549): this compound effectively kills A549 cells upon light activation, and this effect is not influenced by cisplatin resistance [].
- Human adenocarcinoma cells (HT-29): Similar to A549 cells, HT-29 cells are susceptible to this compound-mediated PDT [].
- Human hepatoma cells (PLC/PRF/5): Conjugates of this compound with internalizable ligands exhibit higher photosensitizing activity compared to free this compound, suggesting improved uptake and intracellular targeting [].
- Murine leukemia cells (L1210): Studies with L1210 cells revealed that the phototoxic effects of this compound are distinct from those of hydrophobic photosensitizers, highlighting the importance of its unique localization pattern [].
- Ehrlich ascites tumor (EAT): this compound exhibits selective accumulation and prolonged retention in EAT tumor tissue, leading to significant tumor regression upon photoactivation [].
- B16 melanoma and M-1 sarcoma: Novel amide and boronated amide derivatives of this compound demonstrate superior therapeutic potency compared to this compound in these aggressive tumor models [].
ANone: While not extensively discussed, the research abstracts highlight some safety aspects of this compound:
- Dark toxicity: this compound generally exhibits low dark toxicity, meaning it is minimally toxic to cells in the absence of light activation [, , ].
- Adverse effects: One study mentions severe adverse effects observed when the interval between this compound administration and light exposure was short, highlighting the importance of optimizing treatment parameters [].
ANone: The research highlights several drug delivery and targeting strategies for this compound:
- Conjugation with internalizable ligands: Attaching this compound to ligands recognized by specific cell surface receptors enhances its internalization and improves its delivery to target cells [, , ].
- Encapsulation in nanoparticles: Formulating this compound within nanoparticles, such as liposomes [], micelles [], and nanosuspensions [], improves its solubility, stability, and target specificity.
- Complexation with quantum dots: Combining this compound with quantum dots not only enhances its delivery but also allows for energy transfer, boosting its photodynamic efficacy [, ].
ANone: Various analytical techniques are employed to study this compound and its derivatives:
- Fluorescence Spectroscopy: This method is widely used to investigate the interaction of this compound with proteins [, , ], characterize its photophysical properties [], and study its uptake and distribution in cells and tissues [, , , , ].
- High-Performance Liquid Chromatography (HPLC): HPLC coupled with ultraviolet (UV) detection is used for the quantification of this compound and its derivatives in biological samples, such as plasma and tissues [].
- Electron Spin Resonance (ESR): This technique is employed to study the generation of singlet oxygen by this compound upon light activation [, ].
- Confocal Microscopy: This imaging technique allows for the visualization of this compound distribution within cells and its co-localization with specific organelles [, , ].
ANone: This aspect is not covered in the provided research abstracts.
A: this compound exhibits poor water solubility, which can limit its bioavailability and therapeutic efficacy. This issue is particularly important at physiological pH, where this compound tends to form aggregates, further reducing its solubility and photodynamic activity [].
A: Nanosuspension formulations, using biodegradable sucrose esters as drug carriers, have shown promise in improving the dissolution rate of this compound. This approach increases the surface area of the drug particles, enhancing its dissolution and improving its bioavailability [].
A: The development and validation of a specific, sensitive, and accurate HPLC-UV method for the quantification of the novel this compound derivative, DYSP-C34, in rat plasma and mouse tissues is described in one study []. The method exhibited good linearity, recovery, precision, and stability, meeting the requirements for biological sample analysis.
ANone: This aspect is not covered in the provided research abstracts.
ANone: This aspect is not covered in the provided research abstracts.
ANone: This aspect is not covered in the provided research abstracts.
ANone: This aspect is not covered in the provided research abstracts.
A: While not explicitly addressed, the use of this compound in PDT relies on its biocompatibility, particularly its low dark toxicity. The development of biodegradable drug delivery systems, such as liposomes and nanosuspensions based on natural polymers, further suggests efforts to enhance its biocompatibility and minimize potential long-term effects [, ].
ANone: This aspect is not covered in the provided research abstracts.
ANone: This aspect is not covered in the provided research abstracts.
ANone: The research on this compound exemplifies cross-disciplinary collaboration, encompassing:
- Chemistry: Synthesis and structural modification of this compound derivatives to enhance its photophysical properties and therapeutic efficacy [, , , , ].
- Biology: Understanding the interaction of this compound with cells and tissues, including its uptake, subcellular localization, and mechanisms of phototoxicity [, , , , , , , , , ].
- Medicine: Exploring the clinical application of this compound in PDT for various diseases, including cancer [, , , , , ] and atherosclerosis [].
- Pharmaceutics: Developing and optimizing formulations to improve this compound delivery, solubility, and bioavailability [, , , ].
- Nanotechnology: Utilizing nanomaterials like quantum dots to enhance this compound delivery and photodynamic efficacy [, ].
Descargo de responsabilidad e información sobre productos de investigación in vitro
Tenga en cuenta que todos los artículos e información de productos presentados en BenchChem están destinados únicamente con fines informativos. Los productos disponibles para la compra en BenchChem están diseñados específicamente para estudios in vitro, que se realizan fuera de organismos vivos. Los estudios in vitro, derivados del término latino "in vidrio", involucran experimentos realizados en entornos de laboratorio controlados utilizando células o tejidos. Es importante tener en cuenta que estos productos no se clasifican como medicamentos y no han recibido la aprobación de la FDA para la prevención, tratamiento o cura de ninguna condición médica, dolencia o enfermedad. Debemos enfatizar que cualquier forma de introducción corporal de estos productos en humanos o animales está estrictamente prohibida por ley. Es esencial adherirse a estas pautas para garantizar el cumplimiento de los estándares legales y éticos en la investigación y experimentación.