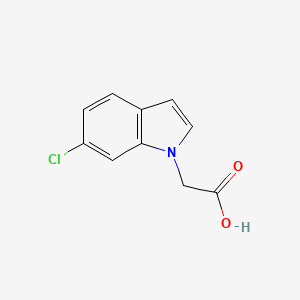
(6-chloro-1H-indol-1-yl)acetic acid
Descripción general
Descripción
“(6-chloro-1H-indol-1-yl)acetic acid” is a chemical compound with the CAS Number: 943654-33-9 . It has a molecular weight of 209.63 and its IUPAC name is this compound . The compound is solid in physical form .
Synthesis Analysis
The synthesis of this compound has been described in various studies . One method involves a novel indole formation that includes an alkylation/1,4-addition/elimination/isomerization cascade .Molecular Structure Analysis
The InChI code for this compound is 1S/C10H8ClNO2/c11-8-2-1-7-3-4-12 (6-10 (13)14)9 (7)5-8/h1-5H,6H2, (H,13,14) .Chemical Reactions Analysis
The compound is a selective cyclooxygenase 2 (COX-2) inhibitor . The synthesis process involves a novel indole formation that includes an alkylation/1,4-addition/elimination/isomerization cascade .Physical And Chemical Properties Analysis
The compound is solid in physical form . It is stored in a dry environment at a temperature between 2-8°C .Aplicaciones Científicas De Investigación
Bacterial Catabolism of Indole-3-acetic Acid
Indole-3-acetic acid (IAA), chemically similar to (6-chloro-1H-indol-1-yl)acetic acid, plays a crucial role in plant growth as a hormone. The study by Laird, Flores, and Leveau (2020) discusses the catabolism of IAA by bacteria. The paper details two gene clusters, iac and iaa, responsible for the aerobic and anaerobic catabolism of IAA, respectively. The significance of these gene clusters extends beyond mere degradation of IAA; they potentially confer benefits to bacterial hosts by utilizing IAA as an energy source or by interfering with IAA-dependent processes in other organisms, such as plants and humans. The paper suggests iac/iaa genes might be pivotal in accessing IAA in diverse environments, including soils, sediments, and even hospital environments, proposing avenues for future research on biotechnological applications and potential treatments for IAA-related pathologies in plants and humans (Laird, Flores, & Leveau, 2020).
Organic Acids in Acidizing Operations
Alhamad, Alrashed, Munif, and Miskimins (2020) provide a comprehensive review of the role of organic acids, including acetic acid, in acidizing operations for carbonate and sandstone formations. The paper underscores the advantages of organic acids over conventional choices like hydrochloric acid, particularly their reduced corrosiveness and better performance in certain conditions. These organic acids are highlighted for their applications in removing formation damage, dissolving drilling mud filter cakes, and serving as iron sequestering agents. This research illustrates the diverse industrial applications of organic acids and their benefits in specific operational scenarios, suggesting a need for further research and development in this area (Alhamad, Alrashed, Munif, & Miskimins, 2020).
Indole Acetic Acid in Plant Growth Under Stress
Ashraf, Azhar, and Hussain (2006) examined the impact of indole acetic acid (IAA) on the growth of barley under water stress conditions. The study revealed that IAA application mitigated the adverse effects of water stress, enhancing plant growth and yield. This indicates the potential of IAA as a growth-promoting agent in agriculture, especially in adverse environmental conditions. The paper's insights into the role of IAA in improving photosynthetic efficiency and growth under stress conditions open up possibilities for its application in agriculture to enhance crop resilience and productivity (Ashraf, Azhar, & Hussain, 2006).
Mecanismo De Acción
Target of Action
It is known that indole derivatives, which include (6-chloro-1h-indol-1-yl)acetic acid, bind with high affinity to multiple receptors . This suggests that the compound could potentially interact with a variety of targets in the body.
Mode of Action
Indole derivatives are known to possess various biological activities, including antiviral, anti-inflammatory, anticancer, anti-hiv, antioxidant, antimicrobial, antitubercular, antidiabetic, antimalarial, and anticholinesterase activities . This suggests that this compound may interact with its targets in a way that modulates these biological activities.
Biochemical Pathways
Given the broad spectrum of biological activities associated with indole derivatives , it is likely that this compound affects multiple biochemical pathways.
Pharmacokinetics
The compound’s molecular weight (20963) and its solid physical form suggest that it may have certain bioavailability characteristics .
Result of Action
The wide range of biological activities associated with indole derivatives suggests that this compound could have diverse effects at the molecular and cellular levels .
Action Environment
Environmental factors can influence the action, efficacy, and stability of this compound. For instance, the compound should be stored in a sealed, dry environment at 2-8°C to maintain its stability . Other environmental factors, such as pH and the presence of other substances, could potentially influence the compound’s action and efficacy.
Safety and Hazards
Análisis Bioquímico
Biochemical Properties
(6-chloro-1H-indol-1-yl)acetic acid plays a crucial role in various biochemical reactions. It interacts with several enzymes, proteins, and other biomolecules, influencing their activity and function. One of the primary interactions of this compound is with the enzyme tryptophan hydroxylase, which is involved in the biosynthesis of serotonin. This interaction can modulate the production of serotonin, thereby affecting mood and behavior. Additionally, this compound has been shown to bind to specific receptors on cell membranes, altering signal transduction pathways and cellular responses .
Cellular Effects
The effects of this compound on various types of cells and cellular processes are significant. In neuronal cells, it influences cell signaling pathways, particularly those involving serotonin and dopamine. This modulation can lead to changes in neurotransmitter release, impacting mood, cognition, and behavior. In cancer cells, this compound has been observed to inhibit cell proliferation and induce apoptosis, making it a potential candidate for anticancer therapies. Furthermore, it affects cellular metabolism by altering the activity of key metabolic enzymes, leading to changes in energy production and utilization .
Molecular Mechanism
The molecular mechanism of action of this compound involves several key processes. At the molecular level, it binds to specific receptors and enzymes, modulating their activity. For instance, its interaction with tryptophan hydroxylase inhibits the enzyme’s activity, reducing serotonin synthesis. Additionally, this compound can activate or inhibit various signaling pathways, leading to changes in gene expression. This modulation of gene expression can result in altered cellular functions, such as increased apoptosis in cancer cells or changes in neurotransmitter release in neuronal cells .
Temporal Effects in Laboratory Settings
In laboratory settings, the effects of this compound change over time. The compound’s stability and degradation are critical factors influencing its long-term effects on cellular function. Studies have shown that this compound remains stable under specific conditions, maintaining its biological activity. Prolonged exposure to light or heat can lead to its degradation, reducing its efficacy. Long-term studies in vitro and in vivo have demonstrated that this compound can have sustained effects on cellular processes, such as continuous inhibition of cell proliferation in cancer cells .
Dosage Effects in Animal Models
The effects of this compound vary with different dosages in animal models. At low doses, the compound has been shown to modulate neurotransmitter levels, leading to changes in behavior and mood. At higher doses, this compound can exhibit toxic effects, such as liver damage and gastrointestinal disturbances. Threshold effects have been observed, where a specific dosage is required to achieve the desired therapeutic effect without causing adverse effects. These findings highlight the importance of dosage optimization in the therapeutic application of this compound .
Metabolic Pathways
This compound is involved in several metabolic pathways. It interacts with enzymes such as tryptophan hydroxylase and monoamine oxidase, influencing the metabolism of neurotransmitters like serotonin and dopamine. These interactions can lead to changes in metabolic flux and metabolite levels, affecting overall cellular metabolism. Additionally, this compound can be metabolized by liver enzymes, leading to the formation of various metabolites that may have distinct biological activities .
Transport and Distribution
The transport and distribution of this compound within cells and tissues are mediated by specific transporters and binding proteins. The compound can cross the blood-brain barrier, allowing it to exert its effects on the central nervous system. Within cells, this compound can bind to intracellular proteins, influencing its localization and accumulation. These interactions can affect the compound’s biological activity and its ability to modulate cellular processes .
Subcellular Localization
The subcellular localization of this compound plays a crucial role in its activity and function. The compound can be targeted to specific cellular compartments, such as the nucleus or mitochondria, through targeting signals or post-translational modifications. This localization can influence its ability to modulate gene expression or cellular metabolism. For example, this compound’s presence in the nucleus can lead to changes in transcriptional activity, while its localization in mitochondria can affect energy production and apoptosis .
Propiedades
IUPAC Name |
2-(6-chloroindol-1-yl)acetic acid | |
---|---|---|
Source | PubChem | |
URL | https://pubchem.ncbi.nlm.nih.gov | |
Description | Data deposited in or computed by PubChem | |
InChI |
InChI=1S/C10H8ClNO2/c11-8-2-1-7-3-4-12(6-10(13)14)9(7)5-8/h1-5H,6H2,(H,13,14) | |
Source | PubChem | |
URL | https://pubchem.ncbi.nlm.nih.gov | |
Description | Data deposited in or computed by PubChem | |
InChI Key |
OUXDBYYTCZPJLG-UHFFFAOYSA-N | |
Source | PubChem | |
URL | https://pubchem.ncbi.nlm.nih.gov | |
Description | Data deposited in or computed by PubChem | |
Canonical SMILES |
C1=CC(=CC2=C1C=CN2CC(=O)O)Cl | |
Source | PubChem | |
URL | https://pubchem.ncbi.nlm.nih.gov | |
Description | Data deposited in or computed by PubChem | |
Molecular Formula |
C10H8ClNO2 | |
Source | PubChem | |
URL | https://pubchem.ncbi.nlm.nih.gov | |
Description | Data deposited in or computed by PubChem | |
Molecular Weight |
209.63 g/mol | |
Source | PubChem | |
URL | https://pubchem.ncbi.nlm.nih.gov | |
Description | Data deposited in or computed by PubChem | |
Retrosynthesis Analysis
AI-Powered Synthesis Planning: Our tool employs the Template_relevance Pistachio, Template_relevance Bkms_metabolic, Template_relevance Pistachio_ringbreaker, Template_relevance Reaxys, Template_relevance Reaxys_biocatalysis model, leveraging a vast database of chemical reactions to predict feasible synthetic routes.
One-Step Synthesis Focus: Specifically designed for one-step synthesis, it provides concise and direct routes for your target compounds, streamlining the synthesis process.
Accurate Predictions: Utilizing the extensive PISTACHIO, BKMS_METABOLIC, PISTACHIO_RINGBREAKER, REAXYS, REAXYS_BIOCATALYSIS database, our tool offers high-accuracy predictions, reflecting the latest in chemical research and data.
Strategy Settings
Precursor scoring | Relevance Heuristic |
---|---|
Min. plausibility | 0.01 |
Model | Template_relevance |
Template Set | Pistachio/Bkms_metabolic/Pistachio_ringbreaker/Reaxys/Reaxys_biocatalysis |
Top-N result to add to graph | 6 |
Feasible Synthetic Routes
Descargo de responsabilidad e información sobre productos de investigación in vitro
Tenga en cuenta que todos los artículos e información de productos presentados en BenchChem están destinados únicamente con fines informativos. Los productos disponibles para la compra en BenchChem están diseñados específicamente para estudios in vitro, que se realizan fuera de organismos vivos. Los estudios in vitro, derivados del término latino "in vidrio", involucran experimentos realizados en entornos de laboratorio controlados utilizando células o tejidos. Es importante tener en cuenta que estos productos no se clasifican como medicamentos y no han recibido la aprobación de la FDA para la prevención, tratamiento o cura de ninguna condición médica, dolencia o enfermedad. Debemos enfatizar que cualquier forma de introducción corporal de estos productos en humanos o animales está estrictamente prohibida por ley. Es esencial adherirse a estas pautas para garantizar el cumplimiento de los estándares legales y éticos en la investigación y experimentación.