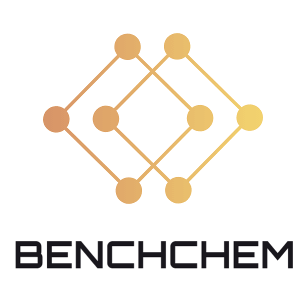
Griseofulvin-13C,d3
- Click on QUICK INQUIRY to receive a quote from our team of experts.
- With the quality product at a COMPETITIVE price, you can focus more on your research.
Overview
Description
Griseofulvin-13C,d3 is the 13C- and deuterium labeled Griseofulvin . It is a stable isotope and has been used in various research studies .
Synthesis Analysis
Griseofulvin is an antifungal polyketide metabolite produced mainly by ascomycetes . The nonreducing polyketide synthase gsfA initiates the synthesis of griseofulvin by combining one acetyl-CoA and six malonyl-CoA units to form the heptaketide backbone of benzophenone . In a recent study, twenty-seven griseofulvin derivatives were synthesized and analyzed .Molecular Structure Analysis
The molecular formula of this compound is C17H17ClO6 . The IUPAC name is (2 S ,5’ R )-7-chloro-3’,6-dimethoxy-5’-methyl-4- (trideuterio (1 13 C)methoxy)spiro [1-benzofuran-2,4’-cyclohex-2-ene]-1’,3-dione .Physical And Chemical Properties Analysis
The molecular weight of this compound is 356.77 g/mol . It has a complexity of 575 and a topological polar surface area of 71.1 Ų .Scientific Research Applications
Multifunctional Applications and Repurposing Potentials
Griseofulvin, including its isotopically labeled forms such as Griseofulvin-13C,d3, is primarily known as an antifungal medication. However, recent studies have expanded its potential applications, particularly in the context of scientific research and development of novel therapeutic interventions. Griseofulvin's ability to disrupt mitosis and cell division in human cancer cells, arrest hepatitis C virus replication, and enhance ACE2 function, suggests its repurposing potential beyond its traditional use. Molecular docking analysis has revealed Griseofulvin and its derivatives, including isotopically labeled versions, to have good binding potential with SARS-CoV-2 main protease, RNA-dependent RNA polymerase (RdRp), and spike protein receptor-binding domain (RBD). This suggests inhibitory effects on SARS-CoV-2 entry and viral replication, highlighting Griseofulvin's multifunctional applications and repurposing potentials in the design and development of novel therapeutic interventions for a range of diseases, including COVID-19 (Aris et al., 2022).
Nanotechnology and Bioavailability Enhancement
The use of nanotechnology to enhance the aqueous solubility and bioavailability of Griseofulvin, including its isotopically labeled forms, represents another significant area of scientific research. Poor water solubility of drugs like Griseofulvin limits their therapeutic applications. However, recent advances in nanotechnology have led to the development of nanoformulations through various techniques such as milling, high-pressure homogenization, and lipid-based formulations. These approaches have shown promise in enhancing the solubility and bioavailability of Griseofulvin, making it more effective as a therapeutic agent. This research not only contributes to the field of drug delivery and pharmacokinetics but also opens new avenues for the application of Griseofulvin in treating diseases where its poor solubility has been a barrier (Kumar, 2019).
Mechanism of Action
Target of Action
Griseofulvin-13C,d3, a labeled form of Griseofulvin , primarily targets fungal microtubules . These microtubules are crucial components of the cell’s cytoskeleton and play a significant role in various cellular processes, including mitosis .
Mode of Action
This compound interacts with its targets by binding to alpha and beta tubulin , the building blocks of microtubules. This binding alters fungal mitosis, inhibiting fungal cell division and nuclear acid synthesis . The compound’s interaction with its targets results in the disruption of the fungal cell’s normal functioning, leading to its eventual death .
Biochemical Pathways
It is known that the compound disrupts mitosis in fungal cells by interfering with microtubule function . In cancer research, Griseofulvin has shown inhibitory effects on cancer cell division and may induce cell death through interaction with the mitotic spindle microtubule . Furthermore, Griseofulvin may inhibit hepatitis C virus replication by interfering with microtubule polymerization in human cells .
Pharmacokinetics
Studies on griseofulvin have shown that its water solubility can be significantly increased through complexation with hp-γ-cyclodextrin . This increase in solubility can enhance the bioavailability of the drug, leading to improved pharmacokinetic profiles . In an in vivo dog pharmacokinetic study, the Cmax was increased from 0.52 µg/mL to 0.72 µg/mL, AUC0–12 was increased from 1.55 μg·h/mL to 2.75 μg·h/mL, the clearance was changed from 51.78 L/kg/h to 24.16 L/kg/h, and the half-life time was changed from 0.81 h to 1.56 h .
Result of Action
The primary result of this compound’s action is the inhibition of fungal growth, making it an effective antifungal agent . By disrupting the function of fungal microtubules, it inhibits fungal cell mitosis, leading to the death of the fungal cell . In addition to its antifungal effects, Griseofulvin has shown potential in cancer research, where it has demonstrated inhibitory effects on cancer cell division .
Safety and Hazards
Griseofulvin-13C,d3 should be used only under a chemical fume hood. Personal protective equipment/face protection should be worn and contact with eyes, skin, or clothing should be avoided . In case of fire, hazardous decomposition products such as carbon oxides (CO, CO2), nitrogen oxides (NOx), and hydrogen chloride gas may be produced .
Future Directions
Griseofulvin has gained increasing interest for multifunctional applications due to its potential to disrupt mitosis and cell division in human cancer cells and arrest hepatitis C virus replication . Molecular docking analysis revealed that griseofulvin and its derivatives have good binding potential with SARS-CoV-2 main protease, RNA-dependent RNA polymerase (RdRp), and spike protein receptor-binding domain (RBD), suggesting its inhibitory effects on SARS-CoV-2 entry and viral replication . These findings imply the repurposing potentials of the FDA-approved drug griseofulvin in designing and developing novel therapeutic interventions .
Biochemical Analysis
Biochemical Properties
Griseofulvin-13C,d3, like its parent compound Griseofulvin, is thought to inhibit fungal cell mitosis and nuclear acid synthesis . It binds to and interferes with the function of spindle and cytoplasmic microtubules by binding to alpha and beta tubulin . This interaction disrupts the mitotic spindle, leading to the production of multinucleate fungal cells .
Cellular Effects
This compound has shown inhibitory effects on cancer cell division and may induce cell death through interaction with the mitotic spindle microtubule . Furthermore, it may inhibit hepatitis C virus replication by interfering with microtubule polymerization in human cells .
Molecular Mechanism
The molecular mechanism of this compound is thought to be similar to that of Griseofulvin. It disrupts mitosis and cell division in human cancer cells and arrests hepatitis C virus replication . Molecular docking analysis revealed that Griseofulvin and its derivatives have good binding potential with SARS-CoV-2 main protease, RNA-dependent RNA polymerase (RdRp), and spike protein receptor-binding domain (RBD), suggesting its inhibitory effects on SARS-CoV-2 entry and viral replication .
Temporal Effects in Laboratory Settings
Griseofulvin has been shown to have long-term effects on cellular function, including disruption of mitosis and cell division .
Metabolic Pathways
Griseofulvin is a mycotoxic metabolic product of Penicillium spp .
Transport and Distribution
Griseofulvin is known to be deposited in keratin precursor cells within 4–8 hours of oral administration .
Subcellular Localization
Griseofulvin has been shown to disrupt the association of connexin 43 with tubulin, leading to an enhanced translocation of connexin 43 from the cytoplasm to the nucleus .
properties
{ "Design of the Synthesis Pathway": "The synthesis of Griseofulvin-13C,d3 involves the incorporation of 13C and deuterium isotopes into the Griseofulvin molecule. This can be achieved through a multi-step synthesis pathway starting from commercially available starting materials.", "Starting Materials": [ "2,4,6-Trichloropyrimidine", "Ethyl acetoacetate", "2-Methyl-6-nitrobenzoic acid", "13C-labeled acetic anhydride", "Deuterium oxide", "Sodium borohydride", "Sodium hydroxide", "Methanol", "Acetone", "Diethyl ether", "Chloroform", "Acetic acid", "Hydrochloric acid", "Sodium chloride" ], "Reaction": [ "Step 1: Synthesis of 2-methyl-6-nitrobenzoyl chloride by reacting 2-methyl-6-nitrobenzoic acid with thionyl chloride in chloroform.", "Step 2: Synthesis of 2-methyl-6-nitrobenzoyl ethyl acetoacetate by reacting 2-methyl-6-nitrobenzoyl chloride with ethyl acetoacetate in the presence of sodium methoxide in methanol.", "Step 3: Synthesis of 2,4,6-trichloropyrimidine-13C,d3 by reacting 2,4,6-trichloropyrimidine with 13C-labeled acetic anhydride and deuterium oxide in the presence of sodium hydroxide in acetone.", "Step 4: Synthesis of Griseofulvin-13C,d3 by reacting 2-methyl-6-nitrobenzoyl ethyl acetoacetate and 2,4,6-trichloropyrimidine-13C,d3 in the presence of sodium borohydride in methanol.", "Step 5: Purification of Griseofulvin-13C,d3 by recrystallization from a mixture of chloroform and methanol.", "Step 6: Final purification of Griseofulvin-13C,d3 by washing with dilute hydrochloric acid and sodium chloride solution, followed by drying." ] } | |
CAS RN |
1329612-29-4 |
Molecular Formula |
C17H17ClO6 |
Molecular Weight |
356.778 |
IUPAC Name |
(2S,5/'R)-7-chloro-3/',6-dimethoxy-5/'-methyl-4-(trideuteriomethoxy)spiro[1-benzofuran-2,4/'-cyclohex-2-ene]-1/',3-dione |
InChI |
InChI=1S/C17H17ClO6/c1-8-5-9(19)6-12(23-4)17(8)16(20)13-10(21-2)7-11(22-3)14(18)15(13)24-17/h6-8H,5H2,1-4H3/t8-,17+/m1/s1/i2+1D3 |
InChI Key |
DDUHZTYCFQRHIY-IQVAYXSPSA-N |
SMILES |
CC1CC(=O)C=C(C12C(=O)C3=C(O2)C(=C(C=C3OC)OC)Cl)OC |
synonyms |
(1’S,6’R)-7-Chloro-2’,6-dimethoxy-4-(methoxy-d3)-6’-methyl-spiro[benzofuran-2(3H),1’-[2]cyclohexene]-3,4’-dione; Amudane-13C,d3; Fulcin-13C,d3; Grisovin-13C,d3; Spirofulvin-13C,d3; Sporostatin-13C,d3; Xuanjing-13C,d3; |
Origin of Product |
United States |
Disclaimer and Information on In-Vitro Research Products
Please be aware that all articles and product information presented on BenchChem are intended solely for informational purposes. The products available for purchase on BenchChem are specifically designed for in-vitro studies, which are conducted outside of living organisms. In-vitro studies, derived from the Latin term "in glass," involve experiments performed in controlled laboratory settings using cells or tissues. It is important to note that these products are not categorized as medicines or drugs, and they have not received approval from the FDA for the prevention, treatment, or cure of any medical condition, ailment, or disease. We must emphasize that any form of bodily introduction of these products into humans or animals is strictly prohibited by law. It is essential to adhere to these guidelines to ensure compliance with legal and ethical standards in research and experimentation.