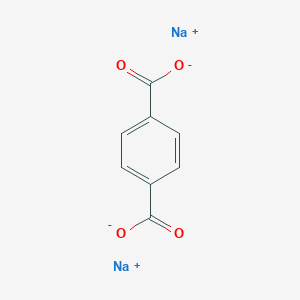
Disodium terephthalate
Overview
Description
Disodium terephthalate belongs to the class of organic salts. It is commonly used as a catalyst or intermediate in the production of a variety of industrial and consumer products, including polyethylene terephthalate (PET) plastics, polyester fibers, and films . It has excellent thermal stability, chemical resistance, and mechanical properties, making it ideal for high-performance applications . Additionally, it is non-toxic, non-flammable, and environmentally friendly .
Synthesis Analysis
Crude this compound is prepared by the depolymerization of PET . The reaction of 10 g polyester, 10 g sodium bicarbonate, and 50 g ethylene glycol at 180°C was studied, resulting in a 99% depolymerization rate and this compound .Molecular Structure Analysis
The molecular formula of this compound is C8H4Na2O4 . Its average mass is 210.094 Da and its monoisotopic mass is 209.990494 Da .Chemical Reactions Analysis
This compound and its various derivatives are synthesized via simple acid-base chemistry for anode materials in Na-ion batteries . They show excellent electrochemical performance, including little capacity fading over 90 cycles, ideal redox potential, and excellent rate performance .Physical And Chemical Properties Analysis
This compound is a white powder . It is soluble in water . The solubility and freezing points decrease with increasing concentrations of ethylene glycol .Scientific Research Applications
Energy Storage:
- Disodium terephthalate is investigated as an organic anode material for sodium-ion batteries. It shows promising electrochemical performance, including little capacity fading over multiple cycles, ideal redox potential, and excellent rate performance (Park et al., 2012).
- Research into sodium storage mechanisms in this compound as an anode for organic batteries reveals insights into how sodium ions interact with the material, significantly impacting battery performance (Mahasin Alam Sk & Manzhos, 2016).
Material Science:
- Spectroscopic studies on this compound, including IR, Raman, and SERS spectra, provide insights into its molecular orientation and interactions, which are crucial for various material science applications (Varghese et al., 2007).
- The study of crystal structures of this compound and related salts contributes to a deeper understanding of their properties, with implications for their use in various applications such as catalysis and adsorption (Kaduk, 2000).
Environmental Applications:
- This compound is a subject of research in microbial bioprocessing. Studies demonstrate its degradation by specific microorganisms, which could have implications for environmental cleanup or recycling processes (Sugimori & Sekiguchi, 2002).
- A model-based operational optimization of microbial bioprocesses converting terephthalic acid to biomass focuses on improving automation and operational efficiency of using this compound for microbial growth (Beagan et al., 2020).
Adsorption and Filtration:
- The application of this compound in nanofiltration processes for the recovery of caustic soda and concentration from wastewater demonstrates its potential in water treatment and industrial processes (Choe et al., 2005).
Mechanism of Action
Target of Action
Disodium terephthalate, an organic salt, is primarily targeted towards both lithium and sodium . It has been widely used in the preparation of organometallic frame compounds, battery materials, and leather production aids .
Mode of Action
The precise mechanism of action of this compound remains partly elusive. It is believed to function as a chelating agent, forming complexes by binding to metal ions . This interaction with metal ions can lead to alterations in the structure and function of proteins, enzymes, and other molecules .
Biochemical Pathways
In the degradation of phthalates, phthalic acid is reported to be the intermediate product. The metabolic pathways and ring cleavage of phthalic acid can be used for further utilization of the phthalic acid generated . Metabolic pathways of phthalic acid in anaerobic and aerobic process conditions are different .
Result of Action
This compound and its various derivatives are synthesized via simple acid-base chemistry for anode materials in sodium-ion batteries. They show excellent electrochemical performance, including little capacity fading over 90 cycles, ideal redox potential, and excellent rate performance, making them promising candidates for sodium-ion batteries .
Action Environment
The inferior cycling stability of this compound as an anode for sodium-ion batteries (SIBs) compared to lithium-ion batteries (LIBs) could be due to the larger ion radius of Na+ than Li+, which results in larger steric resistance and polarization during electrochemical energy storage (EES). Therefore, this compound shows greater changes in spectra during de/sodiation than de/lithiation .
Safety and Hazards
Disodium terephthalate is moderately toxic by the intravenous route . It is advised to avoid dust formation, breathing mist, gas, or vapors, and contacting with skin and eye . Use personal protective equipment, wear chemical impermeable gloves, ensure adequate ventilation, remove all sources of ignition, and evacuate personnel to safe areas .
Biochemical Analysis
Biochemical Properties
Disodium terephthalate is used as a biochemical reagent in life science-related research .
Cellular Effects
Given its role as a pH regulator and buffer in the food and pharmaceutical industries , it may influence cell function by maintaining optimal pH conditions for cellular metabolism.
Molecular Mechanism
It is known to exert its effects at the molecular level, potentially through binding interactions with biomolecules, enzyme inhibition or activation, and changes in gene expression .
Temporal Effects in Laboratory Settings
Given its excellent thermal stability , it is likely to have a stable effect on cellular function over time.
Transport and Distribution
It may interact with transporters or binding proteins, influencing its localization or accumulation .
Subcellular Localization
It may be directed to specific compartments or organelles by targeting signals or post-translational modifications .
properties
{ "Design of the Synthesis Pathway": "Disodium terephthalate can be synthesized by the reaction of terephthalic acid with sodium hydroxide in water.", "Starting Materials": ["Terephthalic acid", "Sodium hydroxide", "Water"], "Reaction": ["1. Dissolve terephthalic acid in water to form a solution.", "2. Add sodium hydroxide to the solution and stir until all the terephthalic acid dissolves.", "3. Heat the solution to 80-90°C and maintain the temperature for 2-3 hours.", "4. Allow the solution to cool and filter the precipitated disodium terephthalate.", "5. Wash the product with water and dry it at 100°C." ] } | |
CAS RN |
10028-70-3 |
Molecular Formula |
C8H6NaO4 |
Molecular Weight |
189.12 g/mol |
IUPAC Name |
disodium;terephthalate |
InChI |
InChI=1S/C8H6O4.Na/c9-7(10)5-1-2-6(4-3-5)8(11)12;/h1-4H,(H,9,10)(H,11,12); |
InChI Key |
IISLNQNUYOZKNE-UHFFFAOYSA-N |
SMILES |
C1=CC(=CC=C1C(=O)[O-])C(=O)[O-].[Na+].[Na+] |
Canonical SMILES |
C1=CC(=CC=C1C(=O)O)C(=O)O.[Na] |
Other CAS RN |
10028-70-3 |
Related CAS |
15596-76-6 |
synonyms |
disodium terephthalate terephthalate terephthalic acid |
Origin of Product |
United States |
Synthesis routes and methods I
Procedure details
Synthesis routes and methods II
Procedure details
Retrosynthesis Analysis
AI-Powered Synthesis Planning: Our tool employs the Template_relevance Pistachio, Template_relevance Bkms_metabolic, Template_relevance Pistachio_ringbreaker, Template_relevance Reaxys, Template_relevance Reaxys_biocatalysis model, leveraging a vast database of chemical reactions to predict feasible synthetic routes.
One-Step Synthesis Focus: Specifically designed for one-step synthesis, it provides concise and direct routes for your target compounds, streamlining the synthesis process.
Accurate Predictions: Utilizing the extensive PISTACHIO, BKMS_METABOLIC, PISTACHIO_RINGBREAKER, REAXYS, REAXYS_BIOCATALYSIS database, our tool offers high-accuracy predictions, reflecting the latest in chemical research and data.
Strategy Settings
Precursor scoring | Relevance Heuristic |
---|---|
Min. plausibility | 0.01 |
Model | Template_relevance |
Template Set | Pistachio/Bkms_metabolic/Pistachio_ringbreaker/Reaxys/Reaxys_biocatalysis |
Top-N result to add to graph | 6 |
Feasible Synthetic Routes
Q & A
Q1: What is the molecular formula and weight of disodium terephthalate?
A1: The molecular formula of this compound is Na2C8H4O4, and its molecular weight is 210.10 g/mol.
Q2: How is this compound characterized spectroscopically?
A2: this compound can be characterized using various spectroscopic techniques, including 1H NMR, which reveals the presence and purity of the terephthalic acid core. [ [] ]
Q3: What is the solubility of this compound in different solvents?
A3: this compound exhibits significant solubility in water and aqueous sodium hydroxide solutions. Its solubility increases with temperature. It also shows solubility in water-ethylene glycol mixtures. [ [] ]
Q4: Can this compound be used as an anode material in sodium-ion batteries?
A5: Yes, this compound has shown promise as a high-performance anode material for low-cost, room-temperature sodium-ion batteries. [ [] ] It exhibits excellent electrochemical performance, including minimal capacity fading over numerous cycles, an ideal redox potential, and excellent rate performance. [ [] ]
Q5: How does the molecular structure of this compound influence its performance as an anode material compared to related compounds?
A6: Density Functional Theory (DFT) calculations suggest that substituting the aromatic ring of this compound with pyridine, as in disodium pyridine dicarboxylate (Na2PDC), can lead to an increased voltage. [ [] ] This highlights the impact of molecular structure modifications on the electrochemical properties of these compounds.
Q6: Can this compound be used in the synthesis of metal-organic frameworks (MOFs)?
A7: Yes, this compound serves as an excellent organic linker for synthesizing various MOFs, including nickel terephthalate. [ [] ] It can be used to create MOFs with unique properties, such as high porosity and enhanced catalytic activity. [ [] ]
Q7: What are the advantages of using this compound as a linker source in MOF synthesis compared to traditional methods?
A8: Utilizing this compound as a linker source allows for green synthesis of MOFs in water at room temperature. [ [] ] This method offers several advantages over conventional approaches, including faster reaction times, lower energy consumption, and reduced environmental impact.
Q8: What is the catalytic activity of nickel terephthalate (Ni-MOF) synthesized using this compound?
A9: Ni-MOF synthesized using this compound exhibits enhanced catalytic efficiency in hydrogen generation from sodium borohydride compared to its metal salt counterpart. [ [] ] This enhanced activity is attributed to the unique structural properties of the MOF.
Q9: How is this compound produced from waste PET?
A10: this compound can be recovered from waste PET through alkaline hydrolysis using sodium hydroxide. [ [, , ] ] This process breaks down PET into its monomers, this compound and ethylene glycol. [ [, , ] ]
Q10: What factors influence the rate of PET depolymerization to this compound?
A11: Several factors influence the depolymerization rate, including temperature, NaOH concentration, PET particle size, catalyst concentration, and the presence of co-solvents like dioxane. [ [, ] ]
Q11: Can this compound be further purified after recovery from PET waste?
A12: Yes, the recovered this compound can be further purified by acidification with sulfuric acid, yielding high-purity terephthalic acid. [ [, , ] ] This purified terephthalic acid can then be used in the production of new PET, closing the recycling loop.
Q12: What are the environmental benefits of recycling PET via this compound recovery?
A12: Recycling PET via this compound recovery offers several environmental benefits:
- Reduced plastic waste: Diverts waste PET from landfills, mitigating pollution. [ [, ] ]
- Resource conservation: Allows for the recovery and reuse of valuable monomers (terephthalic acid and ethylene glycol). [ [, ] ]
- Lower energy consumption: Compared to virgin PET production, recycling PET requires less energy. [ [] ]
- Decreased reliance on fossil fuels: Reduces the need for new raw materials derived from fossil fuels. [ [] ]
Q13: Can microorganisms degrade this compound?
A14: Yes, certain bacterial strains, such as Rhodococcus sp. and Dietzia sp., can utilize this compound as their sole carbon and energy source. [ [, , , ] ] This biodegradation process typically occurs under aerobic conditions. [ [, ] ]
Q14: What are the intermediates involved in the microbial degradation of this compound?
A15: Protocatechuic acid (PC) has been identified as a key intermediate in the microbial degradation pathway of this compound. [ [, ] ]
Q15: How is this compound used to detect hydroxyl radicals?
A16: this compound serves as a fluorescent probe for detecting hydroxyl radicals (•OH) in various systems. [ [, , , ] ] Upon reacting with •OH, it forms the highly fluorescent product 2-hydroxyterephthalic acid, quantifiable via fluorescence spectroscopy. [ [, , ] ]
Q16: What factors can influence the accuracy of hydroxyl radical quantification using this compound?
A17: Factors such as this compound concentration, the presence of dissolved oxygen, and the decomposition of the fluorescent product by reactive species can affect accurate quantification. [ [, ] ]
Q17: What computational methods are employed to study this compound?
A18: DFT calculations are valuable for investigating the interactions of sodium with this compound, predicting voltage changes with structural modifications, and exploring the electronic properties of the molecule. [ [, , ] ]
Disclaimer and Information on In-Vitro Research Products
Please be aware that all articles and product information presented on BenchChem are intended solely for informational purposes. The products available for purchase on BenchChem are specifically designed for in-vitro studies, which are conducted outside of living organisms. In-vitro studies, derived from the Latin term "in glass," involve experiments performed in controlled laboratory settings using cells or tissues. It is important to note that these products are not categorized as medicines or drugs, and they have not received approval from the FDA for the prevention, treatment, or cure of any medical condition, ailment, or disease. We must emphasize that any form of bodily introduction of these products into humans or animals is strictly prohibited by law. It is essential to adhere to these guidelines to ensure compliance with legal and ethical standards in research and experimentation.