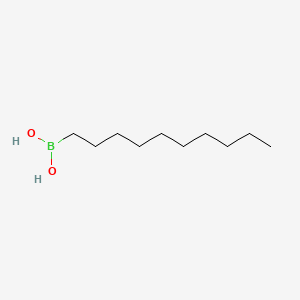
Decylboronic Acid
Overview
Description
Decylboronic Acid, also known as n-Decylboronic acid or Boronic acid, B-decyl-, is a boron-containing compound . It has the molecular formula C10H23BO2 . It is a stable and generally non-toxic group that is easily synthesized .
Synthesis Analysis
Boronic acids, including this compound, are relatively simple and well-known to synthesize . They can be used as building blocks and synthetic intermediates . The introduction of the boronic acid group to bioactive molecules has shown to modify selectivity, physicochemical, and pharmacokinetic characteristics, with the improvement of the already existing activities .
Molecular Structure Analysis
The molecular structure of this compound is represented by the formula C10H23BO2 . It has a molecular weight of 186.10 g/mol .
Chemical Reactions Analysis
Boronic acids, including this compound, are known for their interactions with diols and strong Lewis bases such as fluoride or cyanide anions . This makes them useful in various sensing applications .
Physical and Chemical Properties Analysis
This compound is a white to pale cream crystalline solid . It has a density of 0.9±0.1 g/cm3 . The boiling point is 297.1±23.0 °C at 760 mmHg . It has a molar refractivity of 54.9±0.3 cm3, a polar surface area of 40 Å2, and a molar volume of 210.6±3.0 cm3 .
Scientific Research Applications
Antitumor Effects
Decylboronic acid and related phenylboronic acid derivatives have shown promising antitumor effects. In vitro and in vivo studies demonstrate their cytotoxic activity against various cancer cell lines, including mouse mammary adenocarcinoma and squamous cell carcinoma. The antitumor effects are dose-dependent and notable when administrated intraperitoneally or orally (Marasović et al., 2017). Additionally, phenylboronic acid-modified polyamidoamine has been used for hepatocarcinoma gene therapy, showing potential in disrupting microtubule polymerization, inducing apoptosis, and inhibiting tumor growth (Yang et al., 2019).
Drug Delivery Systems
Phenylboronic acid-based materials have been extensively studied for their application in glucose-responsive drug delivery systems. These include smart drug delivery systems for diabetic therapy, using materials like gels, micelles, capsules, and vesicles (Huang et al., 2019). Moreover, phenylboronic acid-conjugated exosomes have been explored for enhancing anticancer therapeutic effects by increasing drug loading efficiency (Kim et al., 2021).
Targeted Therapy
Phenylboronic acid-decorated nanoparticles have been designed for tumor-targeted drug delivery. These nanoparticles, by conjugating with 3-carboxyphenylboronic acid, enhance tumor-homing activity, thus improving tumor accumulation and antitumor effects (Wang et al., 2016). Furthermore, boronic acid-decorated albumin nanocomposites have been investigated for chemo/herbal therapy in lung cancer, showing promise in localized therapy (Elgohary et al., 2018).
Agricultural Applications
In agriculture, phenylboronic acid derivatives have been studied for their fungicidal properties. They have shown effectiveness in controlling early blight fungus in tomatoes, suggesting their potential as environmentally friendly and efficient antifungal agents (Martinko et al., 2022).
Other Therapeutic Applications
Phenylboronic acid derivatives are being explored for various therapeutic applications, including enzyme-responsive drug delivery for targeted tumor therapy and pH-activated targeting drug delivery systems. These systems are designed to release drugs in response to specific physiological conditions, enhancing therapeutic efficacy while minimizing side effects (Liu et al., 2015), (Zhao et al., 2016).
Mechanism of Action
Target of Action
Decylboronic Acid, also known as n-Decylboronic acid, is primarily used in the Suzuki–Miyaura (SM) cross-coupling reaction . This reaction is a widely-applied transition metal catalysed carbon–carbon bond forming reaction . The primary targets of this compound in this reaction are the organic groups that participate in electronically divergent processes with the metal catalyst .
Mode of Action
The mode of action of this compound involves two key steps: oxidative addition and transmetalation . In the oxidative addition step, palladium becomes oxidized through its donation of electrons to form a new Pd–C bond . In the transmetalation step, this compound, which is formally a nucleophilic organic group, is transferred from boron to palladium .
Biochemical Pathways
It is known that the compound plays a crucial role in the suzuki–miyaura coupling reaction, which is a key process in organic synthesis . The downstream effects of this reaction depend on the specific reactants and conditions used.
Pharmacokinetics
It is known that the compound has a molecular weight of 18610 Da , which may influence its bioavailability and pharmacokinetic properties.
Result of Action
The result of the action of this compound is the formation of a new carbon–carbon bond via the Suzuki–Miyaura coupling reaction . This reaction is widely used in organic synthesis to create a variety of complex organic compounds .
Action Environment
The action, efficacy, and stability of this compound can be influenced by various environmental factors. For instance, the yield of the Suzuki–Miyaura coupling reaction can be increased by slow-release and thus low concentration of boronic acid . .
Safety and Hazards
Decylboronic Acid should be handled with care. Avoid dust formation, breathing mist, gas, or vapors . Avoid contact with skin and eyes . Use personal protective equipment and wear chemical impermeable gloves . Ensure adequate ventilation and remove all sources of ignition . Evacuate personnel to safe areas and keep people away from and upwind of spill/leak .
Biochemical Analysis
Biochemical Properties
Decylboronic acid plays a significant role in biochemical reactions due to its ability to interact with various biomolecules. It forms reversible covalent bonds with diols and polyols, which are commonly found in saccharides and catechols. This interaction is crucial for the molecular recognition processes in biological systems. This compound can also bind to nucleophilic amino acid side chains, such as serine, which allows it to act as an enzyme inhibitor. This property is particularly useful in the design of enzyme inhibitors for therapeutic applications .
Cellular Effects
This compound has been shown to influence various cellular processes. It can affect cell signaling pathways by interacting with proteins and enzymes involved in these pathways. For example, this compound can inhibit the activity of certain kinases, which are enzymes that play a critical role in cell signaling. This inhibition can lead to changes in gene expression and cellular metabolism. Additionally, this compound has been used in the delivery of peptides into cells, demonstrating its ability to cross cell membranes and affect intracellular processes .
Molecular Mechanism
The molecular mechanism of this compound involves its ability to form reversible covalent bonds with diols and nucleophilic amino acid side chains. This interaction allows this compound to inhibit the activity of enzymes by binding to their active sites. The binding of this compound to enzymes can prevent the enzymes from interacting with their natural substrates, thereby inhibiting their activity. This mechanism is particularly useful in the design of enzyme inhibitors for therapeutic applications .
Temporal Effects in Laboratory Settings
In laboratory settings, the effects of this compound can change over time due to its stability and degradation. This compound is relatively stable under physiological conditions, but it can degrade over time, especially in the presence of water. This degradation can lead to a decrease in its effectiveness as an enzyme inhibitor or molecular recognition agent. Long-term studies have shown that this compound can have lasting effects on cellular function, including changes in gene expression and cellular metabolism .
Dosage Effects in Animal Models
The effects of this compound can vary with different dosages in animal models. At low doses, this compound can effectively inhibit enzyme activity and influence cellular processes without causing significant toxicity. At high doses, this compound can cause adverse effects, including toxicity and disruption of normal cellular function. Studies have shown that there is a threshold dose above which the toxic effects of this compound become apparent .
Metabolic Pathways
This compound is involved in various metabolic pathways, primarily through its interaction with enzymes and cofactors. It can affect metabolic flux by inhibiting the activity of key enzymes involved in metabolic pathways. For example, this compound can inhibit the activity of enzymes involved in the glycolytic pathway, leading to changes in metabolite levels and metabolic flux. This inhibition can have downstream effects on cellular metabolism and energy production .
Transport and Distribution
This compound is transported and distributed within cells and tissues through interactions with transporters and binding proteins. It can cross cell membranes and accumulate in specific cellular compartments, such as the cytosol and nucleus. The distribution of this compound within cells can affect its activity and function, as it can interact with different biomolecules in different cellular compartments .
Subcellular Localization
The subcellular localization of this compound is influenced by its ability to interact with targeting signals and post-translational modifications. This compound can be directed to specific compartments or organelles within the cell, such as the endoplasmic reticulum or mitochondria, through interactions with targeting signals. This localization can affect its activity and function, as it can interact with different biomolecules in different subcellular compartments .
Properties
IUPAC Name |
decylboronic acid | |
---|---|---|
Source | PubChem | |
URL | https://pubchem.ncbi.nlm.nih.gov | |
Description | Data deposited in or computed by PubChem | |
InChI |
InChI=1S/C10H23BO2/c1-2-3-4-5-6-7-8-9-10-11(12)13/h12-13H,2-10H2,1H3 | |
Source | PubChem | |
URL | https://pubchem.ncbi.nlm.nih.gov | |
Description | Data deposited in or computed by PubChem | |
InChI Key |
TUTSEMMBKGBUGD-UHFFFAOYSA-N | |
Source | PubChem | |
URL | https://pubchem.ncbi.nlm.nih.gov | |
Description | Data deposited in or computed by PubChem | |
Canonical SMILES |
B(CCCCCCCCCC)(O)O | |
Source | PubChem | |
URL | https://pubchem.ncbi.nlm.nih.gov | |
Description | Data deposited in or computed by PubChem | |
Molecular Formula |
C10H23BO2 | |
Source | PubChem | |
URL | https://pubchem.ncbi.nlm.nih.gov | |
Description | Data deposited in or computed by PubChem | |
DSSTOX Substance ID |
DTXSID70400492 | |
Record name | Decylboronic Acid | |
Source | EPA DSSTox | |
URL | https://comptox.epa.gov/dashboard/DTXSID70400492 | |
Description | DSSTox provides a high quality public chemistry resource for supporting improved predictive toxicology. | |
Molecular Weight |
186.10 g/mol | |
Source | PubChem | |
URL | https://pubchem.ncbi.nlm.nih.gov | |
Description | Data deposited in or computed by PubChem | |
CAS No. |
24464-63-9 | |
Record name | Decylboronic Acid | |
Source | EPA DSSTox | |
URL | https://comptox.epa.gov/dashboard/DTXSID70400492 | |
Description | DSSTox provides a high quality public chemistry resource for supporting improved predictive toxicology. | |
Retrosynthesis Analysis
AI-Powered Synthesis Planning: Our tool employs the Template_relevance Pistachio, Template_relevance Bkms_metabolic, Template_relevance Pistachio_ringbreaker, Template_relevance Reaxys, Template_relevance Reaxys_biocatalysis model, leveraging a vast database of chemical reactions to predict feasible synthetic routes.
One-Step Synthesis Focus: Specifically designed for one-step synthesis, it provides concise and direct routes for your target compounds, streamlining the synthesis process.
Accurate Predictions: Utilizing the extensive PISTACHIO, BKMS_METABOLIC, PISTACHIO_RINGBREAKER, REAXYS, REAXYS_BIOCATALYSIS database, our tool offers high-accuracy predictions, reflecting the latest in chemical research and data.
Strategy Settings
Precursor scoring | Relevance Heuristic |
---|---|
Min. plausibility | 0.01 |
Model | Template_relevance |
Template Set | Pistachio/Bkms_metabolic/Pistachio_ringbreaker/Reaxys/Reaxys_biocatalysis |
Top-N result to add to graph | 6 |
Feasible Synthetic Routes
Disclaimer and Information on In-Vitro Research Products
Please be aware that all articles and product information presented on BenchChem are intended solely for informational purposes. The products available for purchase on BenchChem are specifically designed for in-vitro studies, which are conducted outside of living organisms. In-vitro studies, derived from the Latin term "in glass," involve experiments performed in controlled laboratory settings using cells or tissues. It is important to note that these products are not categorized as medicines or drugs, and they have not received approval from the FDA for the prevention, treatment, or cure of any medical condition, ailment, or disease. We must emphasize that any form of bodily introduction of these products into humans or animals is strictly prohibited by law. It is essential to adhere to these guidelines to ensure compliance with legal and ethical standards in research and experimentation.