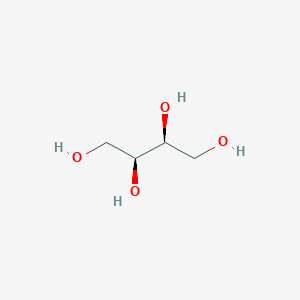
l-Threitol
Overview
Description
D-Treitol is a four-carbon sugar alcohol, also known as a tetritol, with the molecular formula C4H10O4. It is found naturally in certain algae, fungi, and lichens. D-Treitol is known for being twice as sweet as sucrose and has applications as a coronary vasodilator .
Scientific Research Applications
D-Treitol has a wide range of applications in scientific research:
Chemistry: Used as an intermediate in the synthesis of other compounds.
Biology: Acts as an osmoprotective agent in certain osmotolerant yeasts.
Medicine: Investigated for its potential use as a coronary vasodilator.
Industry: Utilized in the production of sugar substitutes and as a cryoprotectant in certain organisms.
Mechanism of Action
D-Treitol exerts its effects primarily through its role as a sugar alcohol. It interacts with various molecular targets, including enzymes involved in carbohydrate metabolism. In biological systems, it acts as an osmoprotectant, helping cells to maintain osmotic balance under stress conditions .
Similar Compounds:
Erythritol: A diastereomer of D-Treitol, used as a sugar substitute.
L-Treitol: The enantiomer of D-Treitol, with similar chemical properties but different biological activities.
Xylitol: Another sugar alcohol with similar applications in food and medicine.
Uniqueness: D-Treitol is unique due to its specific stereochemistry, which influences its sweetness and biological activity. Unlike erythritol, D-Treitol is not commonly used as a sugar substitute but has specialized applications in medicine and research .
Safety and Hazards
Future Directions
Biochemical Analysis
Biochemical Properties
L-Threitol plays a significant role in biochemical reactions, particularly in the context of metabolic pathways involving sugar alcohols. It interacts with various enzymes and proteins, including xylitol dehydrogenase, which catalyzes the conversion of erythritol to this compound. This interaction is crucial for the biosynthesis of this compound in microbial cell factories. Additionally, this compound can act as a substrate for other dehydrogenases and reductases, participating in redox reactions that are essential for cellular metabolism .
Cellular Effects
This compound influences various types of cells and cellular processes. It has been observed to affect cell signaling pathways, gene expression, and cellular metabolism. For instance, this compound can modulate the activity of certain signaling molecules and transcription factors, leading to changes in gene expression patterns. This, in turn, can impact cellular functions such as growth, differentiation, and stress responses. Moreover, this compound’s involvement in redox reactions can influence cellular energy metabolism and the balance of reactive oxygen species .
Molecular Mechanism
The molecular mechanism of this compound involves its interactions with specific biomolecules, including enzymes and proteins. This compound can bind to the active sites of dehydrogenases and reductases, facilitating redox reactions that are critical for cellular metabolism. Additionally, this compound may act as an inhibitor or activator of certain enzymes, thereby modulating their activity and influencing metabolic pathways. Changes in gene expression induced by this compound can also contribute to its molecular mechanism of action .
Temporal Effects in Laboratory Settings
In laboratory settings, the effects of this compound can change over time. Studies have shown that this compound is relatively stable under standard storage conditions, but its stability can be affected by factors such as temperature, pH, and exposure to light. Over time, this compound may undergo degradation, leading to changes in its biochemical properties and effects on cellular function. Long-term studies in vitro and in vivo have indicated that this compound can have sustained effects on cellular metabolism and gene expression, although the extent of these effects may vary depending on the experimental conditions .
Dosage Effects in Animal Models
The effects of this compound vary with different dosages in animal models. At low to moderate doses, this compound has been shown to have beneficial effects on metabolic health, including improved glucose tolerance and reduced oxidative stress. At high doses, this compound may exhibit toxic or adverse effects, such as liver damage and disruptions in metabolic homeostasis. These threshold effects highlight the importance of careful dosage optimization in experimental studies involving this compound .
Metabolic Pathways
This compound is involved in several metabolic pathways, particularly those related to sugar alcohol metabolism. It is synthesized from erythritol through the action of xylitol dehydrogenase and can be further metabolized by other dehydrogenases and reductases. This compound’s involvement in these pathways can influence metabolic flux and the levels of various metabolites, thereby impacting overall cellular metabolism. Additionally, this compound may interact with cofactors such as NADH and NADPH, which are essential for redox reactions .
Transport and Distribution
Within cells and tissues, this compound is transported and distributed through specific transporters and binding proteins. These transporters facilitate the uptake and efflux of this compound, ensuring its proper localization and accumulation within cells. The distribution of this compound can also be influenced by factors such as tissue type, cellular compartmentalization, and the presence of other metabolites. Understanding the transport and distribution of this compound is crucial for elucidating its physiological roles and potential therapeutic applications .
Subcellular Localization
This compound’s subcellular localization is determined by targeting signals and post-translational modifications that direct it to specific compartments or organelles. For example, this compound may be localized to the cytoplasm, mitochondria, or other organelles where it can participate in metabolic reactions. The subcellular localization of this compound can affect its activity and function, as well as its interactions with other biomolecules. Studies on the subcellular distribution of this compound can provide insights into its role in cellular metabolism and regulation .
Preparation Methods
Synthetic Routes and Reaction Conditions: D-Treitol can be synthesized from erythritol using xylitol dehydrogenase, with erythrulose as an intermediate. This method involves the enzymatic conversion of erythritol to erythrulose, which is then further reduced to D-Treitol .
Industrial Production Methods: An optimized synthetic route involves a five-step procedure starting from tartaric acid. This method includes the use of modern reagents and enhanced work-up conditions to achieve high yields of enantiopure D-Treitol .
Chemical Reactions Analysis
Types of Reactions: D-Treitol undergoes various chemical reactions, including:
Oxidation: D-Treitol can be oxidized to form corresponding aldehydes or ketones.
Reduction: It can be reduced to form simpler alcohols.
Substitution: Hydroxyl groups in D-Treitol can be substituted with other functional groups.
Common Reagents and Conditions:
Oxidation: Common oxidizing agents include potassium permanganate and chromium trioxide.
Reduction: Sodium borohydride and lithium aluminum hydride are typical reducing agents.
Substitution: Acidic or basic catalysts are often used to facilitate substitution reactions.
Major Products:
Oxidation: Aldehydes and ketones.
Reduction: Simpler alcohols.
Substitution: Various substituted derivatives depending on the reagents used.
properties
IUPAC Name |
(2S,3S)-butane-1,2,3,4-tetrol | |
---|---|---|
Source | PubChem | |
URL | https://pubchem.ncbi.nlm.nih.gov | |
Description | Data deposited in or computed by PubChem | |
InChI |
InChI=1S/C4H10O4/c5-1-3(7)4(8)2-6/h3-8H,1-2H2/t3-,4-/m0/s1 | |
Source | PubChem | |
URL | https://pubchem.ncbi.nlm.nih.gov | |
Description | Data deposited in or computed by PubChem | |
InChI Key |
UNXHWFMMPAWVPI-IMJSIDKUSA-N | |
Source | PubChem | |
URL | https://pubchem.ncbi.nlm.nih.gov | |
Description | Data deposited in or computed by PubChem | |
Canonical SMILES |
C(C(C(CO)O)O)O | |
Source | PubChem | |
URL | https://pubchem.ncbi.nlm.nih.gov | |
Description | Data deposited in or computed by PubChem | |
Isomeric SMILES |
C([C@@H]([C@H](CO)O)O)O | |
Source | PubChem | |
URL | https://pubchem.ncbi.nlm.nih.gov | |
Description | Data deposited in or computed by PubChem | |
Molecular Formula |
C4H10O4 | |
Source | PubChem | |
URL | https://pubchem.ncbi.nlm.nih.gov | |
Description | Data deposited in or computed by PubChem | |
Molecular Weight |
122.12 g/mol | |
Source | PubChem | |
URL | https://pubchem.ncbi.nlm.nih.gov | |
Description | Data deposited in or computed by PubChem | |
Physical Description |
White crystalline solid; [Sigma-Aldrich MSDS] | |
Record name | Threitol | |
Source | Haz-Map, Information on Hazardous Chemicals and Occupational Diseases | |
URL | https://haz-map.com/Agents/17999 | |
Description | Haz-Map® is an occupational health database designed for health and safety professionals and for consumers seeking information about the adverse effects of workplace exposures to chemical and biological agents. | |
Explanation | Copyright (c) 2022 Haz-Map(R). All rights reserved. Unless otherwise indicated, all materials from Haz-Map are copyrighted by Haz-Map(R). No part of these materials, either text or image may be used for any purpose other than for personal use. Therefore, reproduction, modification, storage in a retrieval system or retransmission, in any form or by any means, electronic, mechanical or otherwise, for reasons other than personal use, is strictly prohibited without prior written permission. | |
CAS RN |
2319-57-5, 7493-90-5 | |
Record name | 1,2,3,4-Butanetetrol | |
Source | European Chemicals Agency (ECHA) | |
URL | https://echa.europa.eu/information-on-chemicals | |
Description | The European Chemicals Agency (ECHA) is an agency of the European Union which is the driving force among regulatory authorities in implementing the EU's groundbreaking chemicals legislation for the benefit of human health and the environment as well as for innovation and competitiveness. | |
Explanation | Use of the information, documents and data from the ECHA website is subject to the terms and conditions of this Legal Notice, and subject to other binding limitations provided for under applicable law, the information, documents and data made available on the ECHA website may be reproduced, distributed and/or used, totally or in part, for non-commercial purposes provided that ECHA is acknowledged as the source: "Source: European Chemicals Agency, http://echa.europa.eu/". Such acknowledgement must be included in each copy of the material. ECHA permits and encourages organisations and individuals to create links to the ECHA website under the following cumulative conditions: Links can only be made to webpages that provide a link to the Legal Notice page. | |
Record name | 1,2,3,4-Butanetetrol | |
Source | European Chemicals Agency (ECHA) | |
URL | https://echa.europa.eu/information-on-chemicals | |
Description | The European Chemicals Agency (ECHA) is an agency of the European Union which is the driving force among regulatory authorities in implementing the EU's groundbreaking chemicals legislation for the benefit of human health and the environment as well as for innovation and competitiveness. | |
Explanation | Use of the information, documents and data from the ECHA website is subject to the terms and conditions of this Legal Notice, and subject to other binding limitations provided for under applicable law, the information, documents and data made available on the ECHA website may be reproduced, distributed and/or used, totally or in part, for non-commercial purposes provided that ECHA is acknowledged as the source: "Source: European Chemicals Agency, http://echa.europa.eu/". Such acknowledgement must be included in each copy of the material. ECHA permits and encourages organisations and individuals to create links to the ECHA website under the following cumulative conditions: Links can only be made to webpages that provide a link to the Legal Notice page. | |
Retrosynthesis Analysis
AI-Powered Synthesis Planning: Our tool employs the Template_relevance Pistachio, Template_relevance Bkms_metabolic, Template_relevance Pistachio_ringbreaker, Template_relevance Reaxys, Template_relevance Reaxys_biocatalysis model, leveraging a vast database of chemical reactions to predict feasible synthetic routes.
One-Step Synthesis Focus: Specifically designed for one-step synthesis, it provides concise and direct routes for your target compounds, streamlining the synthesis process.
Accurate Predictions: Utilizing the extensive PISTACHIO, BKMS_METABOLIC, PISTACHIO_RINGBREAKER, REAXYS, REAXYS_BIOCATALYSIS database, our tool offers high-accuracy predictions, reflecting the latest in chemical research and data.
Strategy Settings
Precursor scoring | Relevance Heuristic |
---|---|
Min. plausibility | 0.01 |
Model | Template_relevance |
Template Set | Pistachio/Bkms_metabolic/Pistachio_ringbreaker/Reaxys/Reaxys_biocatalysis |
Top-N result to add to graph | 6 |
Feasible Synthetic Routes
Q & A
A: l-Threitol has the molecular formula C4H10O4 and a molecular weight of 122.12 g/mol. [, ]
A: Yes, researchers have used various spectroscopic techniques to characterize this compound, including nuclear magnetic resonance (NMR) spectroscopy to study its conformation in aqueous solution. [] 1H NMR spectra have also been used to confirm the structure of synthesized derivatives. []
A: this compound derivatives have been successfully incorporated into polymers, demonstrating compatibility with materials like poly(butylene succinate) (PBS) and poly(butylene terephthalate) (PBT). [, , ]
A: Studies show that incorporating this compound derivatives into PBS and PBT can influence their thermal and mechanical properties. For instance, it can increase glass transition temperatures, lower melt temperatures, and alter crystallinity. [, ]
A: While not directly catalytic, this compound derivatives like lariat ethers incorporating a monoaza-15-crown-5 unit have been successfully used as phase transfer catalysts in asymmetric Michael addition reactions. []
A: The this compound-based crown ethers have shown good to excellent enantioselectivities in reactions like the addition of 2-nitropropane to trans-chalcone and the reaction of diethyl acetamidomalonate with β-nitrostyrene. []
A: Yes, molecular dynamics simulations have been employed to investigate the conformation of this compound in aqueous solution, especially in relation to its sweetness properties. [] Density functional theory (DFT) calculations have also been used to understand the energetics and stability of its phenylboronic acid esters. []
A: Molecular dynamics simulations suggest that the "sweetness triangle" conformation found in this compound contributes to its sweetness, though it is predicted to be a weaker sweetener than erythritol. []
A: The substituents on the chalcone significantly impact the yield and enantioselectivity in Michael addition reactions catalyzed by this compound-based crown ethers, highlighting the importance of structural modifications for optimizing catalytic activity. []
ANone: The provided research primarily focuses on the chemical synthesis and properties of this compound and its derivatives, with limited information available regarding SHE regulations, environmental impact, or degradation pathways.
A: Derivatives like 1,4-dideoxy-1,4-imino-l-threitol have been shown to act as competitive inhibitors of α-l-arabinofuranosidase III in Monilinia fructigena, highlighting the potential for biological activity within this class of compounds. []
A: Researchers utilize a combination of techniques including NMR spectroscopy, gas chromatography/mass spectrometry (GC/MS), and high-performance liquid chromatography (HPLC) for the analysis of this compound and its derivatives. [, , ]
A: More recent research explores its applications in various fields. This includes its use as a building block for chiral auxiliaries in asymmetric synthesis, as a monomer for the preparation of biodegradable polymers, and as a starting material for the synthesis of bioactive compounds. [, , , ]
Disclaimer and Information on In-Vitro Research Products
Please be aware that all articles and product information presented on BenchChem are intended solely for informational purposes. The products available for purchase on BenchChem are specifically designed for in-vitro studies, which are conducted outside of living organisms. In-vitro studies, derived from the Latin term "in glass," involve experiments performed in controlled laboratory settings using cells or tissues. It is important to note that these products are not categorized as medicines or drugs, and they have not received approval from the FDA for the prevention, treatment, or cure of any medical condition, ailment, or disease. We must emphasize that any form of bodily introduction of these products into humans or animals is strictly prohibited by law. It is essential to adhere to these guidelines to ensure compliance with legal and ethical standards in research and experimentation.