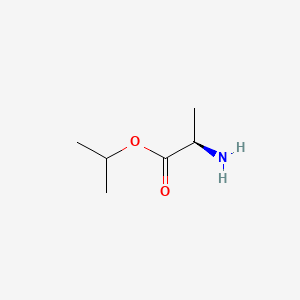
D-Alanine isopropyl ester
Overview
Description
D-Alanine isopropyl ester is a derivative of the amino acid D-alanine. While the provided papers do not directly discuss this compound, they do provide insights into similar compounds and their properties, which can be extrapolated to understand the subject of interest. D-alanine and its derivatives are known for their presence in both invertebrates and vertebrates and have been studied for their physiological relevance and implications in disease .
Synthesis Analysis
The synthesis of D-alanine derivatives can be complex, involving multiple steps and the potential for isomer formation. For example, the synthesis of N-(methoxyacetyl)-N-[2-methyl-6-(methylthio)phenyl]-D,L-alanine methyl ester was carried out in two steps from a related aniline compound, resulting in rotationally hindered isomers known as atropisomers . Similarly, the synthesis of β-benzamido-alpha-(3-pyridyl)-DL-alpha-alanine hydrochloride involved multiple steps, including the formation of an azlactone, hydrolysis to acrylic acid, and subsequent hydrogenation . These processes highlight the complexity and precision required in synthesizing D-alanine derivatives.
Molecular Structure Analysis
The molecular structure of D-alanine derivatives can be analyzed using various spectroscopic methods. For instance, the rotational spectrum of N-acetyl alanine methyl ester was measured to determine the conformational structures of peptide mimetics . This study revealed torsion-rotation splittings and torsional barriers that are critical for understanding the molecular structure. Additionally, the molecular structure, packing properties, and intermolecular interactions of L-alanine alkyl esters were determined using single crystal X-ray diffraction, suggesting that similar techniques could be applied to this compound .
Chemical Reactions Analysis
D-alanine derivatives can participate in various chemical reactions. The biosynthesis of D-alanyl-lipoteichoic acid involves the incorporation of D-alanine into a membrane acceptor, indicating that D-alanine can form ester linkages with other molecules . This reactivity is essential for understanding how D-alanine derivatives might behave in chemical reactions and could provide insights into the potential reactions of this compound.
Physical and Chemical Properties Analysis
The physical and chemical properties of D-alanine derivatives can vary widely. For example, the self-assembly, supramolecular organization, and phase behavior of L-alanine alkyl esters were characterized, revealing critical information about their critical micelle concentrations, transition temperatures, and phase transitions . These properties are influenced by the length of the alkyl chain and the presence of functional groups, which would also be relevant for this compound. Additionally, the fungicidal activity of certain D-alanine derivatives against specific pathogens indicates that these compounds can have significant biological effects .
Scientific Research Applications
Thermal-Responsive Polymers
- Application : D-Alanine isopropyl ester plays a role in the creation of thermal-responsive polymers. These polymers, when synthesized with different side-chain terminal groups, exhibit varied thermal behaviors in water. Notably, replacing methyl ester with isopropyl ester groups in such polymers leads to the loss of thermal-responsive properties (Yu et al., 2014).
Biocatalysis in Medicine and Cosmetics
- Application : Esterase derived from Bacillus cereus, when expressed in E. coli, demonstrates high hydrolytic activity and stereoselectivity towards N-acetyl-DL-alanine methyl ester. This process has applications in producing compounds used in medicine, food, additives, and cosmetics (Zheng et al., 2018).
Gas Chromatography in Analytical Chemistry
- Application : The enantiomeric separation of isopropyl esters of various amino acids, including alanine, is influenced by acylation. This has applications in analytical chemistry, specifically in the area of gas chromatography (Lou et al., 1992).
Autolysin Inhibition in Bacterial Studies
- Application : The D-alanine ester of lipoteichoic acids (LTAs) influences their immunological properties and binding capacity, which is crucial in studying bacterial behaviors, particularly in Staphylococcus aureus (Fischer et al., 1981).
Incorporation into Bacterial Membranes
- Application : D-alanine esters, when incorporated into bacterial membranes, play a critical role in bacterial physiology. This has implications in microbiological research, especially in the study of membrane acceptor ligases in bacteria like Lactobacillus casei (Reusch & Neuhaus, 1971).
Chiral Discrimination in Nanotechnology
- Application : Modified carbon nanotubes with L-alanine ethyl ester exhibit the ability to discriminate between D- and L-tryptophan, showing potential in nanotechnology and electrochemical applications (Kang et al., 2010).
Bacterial Wall Modification
- Application : In Bacillus subtilis, the Dlt operon, responsible for D-alanine esterification in lipoteichoic acid (LTA) and wall teichoic acid (WTA), is crucial for understanding bacterial wall modifications. This is significant in microbiological and biochemical research (Perego et al., 1995).
Safety and Hazards
Mechanism of Action
Target of Action
D-Alanine isopropyl ester primarily targets enzymes such as Thermolysin and D-alanyl-D-alanine carboxypeptidase . These enzymes play a crucial role in bacterial physiology, particularly in the synthesis of the peptidoglycan layer of the bacterial cell wall .
Mode of Action
The mode of action of this compound involves its interaction with these target enzymes. As an ester, it undergoes hydrolysis, a reaction catalyzed by these enzymes, resulting in the release of D-Alanine . D-Alanine is a key constituent of the peptidoglycan layer in bacteria, contributing to the structural integrity of the bacterial cell wall .
Biochemical Pathways
This compound affects the biochemical pathways involved in bacterial cell wall synthesis. The hydrolysis of the ester leads to the production of D-Alanine, which is incorporated into the peptidoglycan layer of the bacterial cell wall . This process is part of a larger biochemical pathway known as the shikimate pathway, which is essential for the biosynthesis of aromatic compounds in bacteria .
Result of Action
The hydrolysis of this compound and the subsequent incorporation of D-Alanine into the peptidoglycan layer can have significant effects at the molecular and cellular levels. It can influence the structural integrity of the bacterial cell wall, potentially affecting bacterial growth and viability .
Action Environment
The action of this compound can be influenced by various environmental factors. For instance, the rate of ester hydrolysis can be affected by factors such as pH and temperature . Additionally, the compound should be stored in a well-ventilated place and kept tightly closed to maintain its stability .
Biochemical Analysis
Biochemical Properties
D-Alanine isopropyl ester plays a significant role in biochemical reactions, particularly in the synthesis of nucleotide prodrugs and synthetic sweeteners . It interacts with various enzymes and proteins, including alanine racemase and D-amino acid oxidase. Alanine racemase catalyzes the conversion of L-alanine to D-alanine, while D-amino acid oxidase is involved in the oxidative deamination of D-alanine. These interactions are crucial for the compound’s role in metabolic pathways and its potential therapeutic applications.
Cellular Effects
This compound has been shown to influence various cellular processes. It affects cell signaling pathways, gene expression, and cellular metabolism. For instance, this compound can modulate the activity of N-methyl-D-aspartate (NMDA) receptors, which are involved in cell signaling and neuroprotection . Additionally, it has been observed to protect tubular epithelial cells from hypoxia-related injury by inhibiting reactive oxygen species production and improving mitochondrial membrane potential .
Molecular Mechanism
The molecular mechanism of this compound involves its interactions with specific biomolecules and enzymes. It binds to NMDA receptors, leading to the activation of signaling pathways that protect cells from oxidative stress . Furthermore, this compound can undergo reductive amination, a process where it forms an imine with an aldehyde or ketone, which is then reduced to an amine . This reaction is essential for the synthesis of various compounds and contributes to the compound’s biochemical properties.
Temporal Effects in Laboratory Settings
In laboratory settings, the effects of this compound can change over time. The compound is relatively stable under standard storage conditions but may degrade under extreme conditions . Long-term studies have shown that this compound can have sustained protective effects on cellular function, particularly in models of acute kidney injury . These temporal effects are important for understanding the compound’s potential therapeutic applications and stability in various experimental settings.
Dosage Effects in Animal Models
The effects of this compound vary with different dosages in animal models. At low doses, the compound has been shown to have protective effects on kidney function and reduce oxidative stress . At higher doses, it may exhibit toxic effects, including potential damage to cellular structures and disruption of metabolic processes . Understanding the dosage effects is crucial for determining the therapeutic window and safety profile of this compound.
Metabolic Pathways
This compound is involved in several metabolic pathways, including those related to amino acid metabolism and nucleotide synthesis . It interacts with enzymes such as alanine racemase and D-amino acid oxidase, which play key roles in the metabolism of D-alanine. These interactions can affect metabolic flux and the levels of various metabolites, highlighting the compound’s importance in cellular metabolism.
Transport and Distribution
Within cells and tissues, this compound is transported and distributed through specific transporters and binding proteins . These transporters facilitate the compound’s movement across cellular membranes and its accumulation in specific cellular compartments. The distribution of this compound can influence its activity and effectiveness in various biological processes.
Subcellular Localization
This compound is localized in specific subcellular compartments, where it exerts its effects on cellular function . It may be directed to particular organelles through targeting signals or post-translational modifications. The subcellular localization of this compound is essential for its role in cellular metabolism and its interactions with other biomolecules.
properties
IUPAC Name |
propan-2-yl (2R)-2-aminopropanoate | |
---|---|---|
Source | PubChem | |
URL | https://pubchem.ncbi.nlm.nih.gov | |
Description | Data deposited in or computed by PubChem | |
InChI |
InChI=1S/C6H13NO2/c1-4(2)9-6(8)5(3)7/h4-5H,7H2,1-3H3/t5-/m1/s1 | |
Source | PubChem | |
URL | https://pubchem.ncbi.nlm.nih.gov | |
Description | Data deposited in or computed by PubChem | |
InChI Key |
QDQVXVRZVCTVHE-RXMQYKEDSA-N | |
Source | PubChem | |
URL | https://pubchem.ncbi.nlm.nih.gov | |
Description | Data deposited in or computed by PubChem | |
Canonical SMILES |
CC(C)OC(=O)C(C)N | |
Source | PubChem | |
URL | https://pubchem.ncbi.nlm.nih.gov | |
Description | Data deposited in or computed by PubChem | |
Isomeric SMILES |
C[C@H](C(=O)OC(C)C)N | |
Source | PubChem | |
URL | https://pubchem.ncbi.nlm.nih.gov | |
Description | Data deposited in or computed by PubChem | |
Molecular Formula |
C6H13NO2 | |
Source | PubChem | |
URL | https://pubchem.ncbi.nlm.nih.gov | |
Description | Data deposited in or computed by PubChem | |
Molecular Weight |
131.17 g/mol | |
Source | PubChem | |
URL | https://pubchem.ncbi.nlm.nih.gov | |
Description | Data deposited in or computed by PubChem | |
Retrosynthesis Analysis
AI-Powered Synthesis Planning: Our tool employs the Template_relevance Pistachio, Template_relevance Bkms_metabolic, Template_relevance Pistachio_ringbreaker, Template_relevance Reaxys, Template_relevance Reaxys_biocatalysis model, leveraging a vast database of chemical reactions to predict feasible synthetic routes.
One-Step Synthesis Focus: Specifically designed for one-step synthesis, it provides concise and direct routes for your target compounds, streamlining the synthesis process.
Accurate Predictions: Utilizing the extensive PISTACHIO, BKMS_METABOLIC, PISTACHIO_RINGBREAKER, REAXYS, REAXYS_BIOCATALYSIS database, our tool offers high-accuracy predictions, reflecting the latest in chemical research and data.
Strategy Settings
Precursor scoring | Relevance Heuristic |
---|---|
Min. plausibility | 0.01 |
Model | Template_relevance |
Template Set | Pistachio/Bkms_metabolic/Pistachio_ringbreaker/Reaxys/Reaxys_biocatalysis |
Top-N result to add to graph | 6 |
Feasible Synthetic Routes
Q & A
Q1: What is the role of D-alanine isopropyl ester in the synthesis of sofosbuvir?
A1: this compound serves as a crucial building block in the multi-step synthesis of sofosbuvir. [] The research article describes its reaction with phenyl dichlorophosphate, followed by treatment with pentafluorophenol and an alkali, to yield a key intermediate compound (formula II in the paper). This intermediate is then further reacted to ultimately produce sofosbuvir.
Q2: The article mentions recycling pentafluorophenyl to minimize environmental impact. How does this recycling occur in the context of this compound's role?
A2: While this compound itself isn't directly involved in the pentafluorophenyl recycling, its use in the synthesis pathway enables this environmentally beneficial process. [] The paper describes how, after the formation of sofosbuvir, the pentafluorophenyl group is cleaved and recovered. This recovered pentafluorophenyl is then reused in the synthesis of the intermediate compound, which, as mentioned earlier, utilizes this compound. This recycling loop reduces waste and minimizes the environmental impact associated with pentafluorophenyl.
Disclaimer and Information on In-Vitro Research Products
Please be aware that all articles and product information presented on BenchChem are intended solely for informational purposes. The products available for purchase on BenchChem are specifically designed for in-vitro studies, which are conducted outside of living organisms. In-vitro studies, derived from the Latin term "in glass," involve experiments performed in controlled laboratory settings using cells or tissues. It is important to note that these products are not categorized as medicines or drugs, and they have not received approval from the FDA for the prevention, treatment, or cure of any medical condition, ailment, or disease. We must emphasize that any form of bodily introduction of these products into humans or animals is strictly prohibited by law. It is essential to adhere to these guidelines to ensure compliance with legal and ethical standards in research and experimentation.