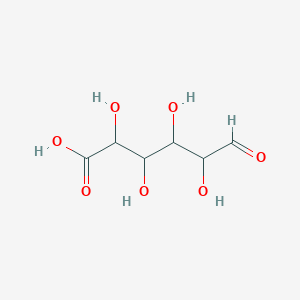
(2S,3R,4S,5R)-2,3,4,5-tetrahydroxy-6-oxohexanoic acid
Overview
Description
(2S,3R,4S,5R)-2,3,4,5-Tetrahydroxy-6-oxohexanoic acid, also known as THOH, is a naturally occurring organic compound that is found in a wide variety of organisms, including plants, animals, and microorganisms. It is a component of the Krebs cycle and is involved in a number of metabolic processes, including energy production, fatty acid synthesis, and amino acid metabolism. THOH has a variety of applications in the scientific community, including its use as a reagent in organic synthesis, a substrate for enzyme assays, and a tool for studying the structure and function of proteins.
Scientific Research Applications
Synthesis of derivatives for isolation of optically pure didemnins. These derivatives, such as (2S,4S)-4-hydroxy-2,5-dimethyl-3-oxohexanoic acid, are important in the context of marine natural products (Gonzalez et al., 1996).
Creation of (2S,5R)-5-hydroxylysine and related alpha-amino acids, useful in the synthesis of amino acids unique to collagen and collagen-like proteins (Marin et al., 2002).
Methyl 5S-(benzoyloxy)-6-oxohexanoate is key in leukotriene synthesis, obtained from 2-cyclohexen-1-one using Schreiber's unsymmetrical ozonolysis protocol (Hayes & Wallace, 1990).
Potential applications in plant growth regulation through the synthesis of optically active cyclohexanone analogs of abscisic acid, dihydroabscisic acids, and their derivatives (Lamb & Abrams, 1990).
Whole cell biosynthesis in organic solvents and biphasic media for the production of key intermediates like tert-Butyl (3R,5S)-6-chloro-3,5-dihydroxyhexanoate, crucial for drugs such as atorvastatin and rosuvastatin (Liu et al., 2018).
Preparation of 6-aroyl-4-oxohexanoic acids with potential anti-inflammatory properties and utility as intermediates for furan synthesis (Short & Rockwood, 1969).
Asymmetric hydrogenation of methyl 3,5-dioxohexanoate with ruthenium catalysts for efficient synthesis of (S)-6-methyl-5,6-dihydro-2-pyrone (Blandin, Carpentier & Mortreux, 1999).
Investigation of unusual fragmentation behavior of 5-oxohexanoic acid in mass spectrometric studies (Kanawati et al., 2007).
Study and application of KmAKR-W297H, a novel NADPH-specific aldo-keto reductase, for improved diastereoselectivity and activity in chemical reactions (Wang et al., 2017).
Applications in enzymatic synthesis for creating tailored enzymes, such as in the preparation of influenza A sialidase inhibitor analogues (Woodhall et al., 2005).
Safety and Hazards
Mechanism of Action
Target of Action
DL-Galacturonic acid is an oxidized form of D-galactose and is the main component of pectin . It primarily targets pectin-rich residues, which accumulate when sugar is extracted from sugar beet or juices are produced from citrus fruits .
Mode of Action
DL-Galacturonic acid interacts with its targets by forming long chains through the hydroxyl groups at C1 and C4 . In its open form, it has an aldehyde group at C1 and a carboxylic acid group at C6 . This interaction results in the formation of polygalacturonic acid, a polymer of DL-Galacturonic acid .
Biochemical Pathways
DL-Galacturonic acid is involved in the catabolism of D-galacturonic acid, which is relevant for the microbial conversion to useful products . An alternative bacterial pathway exists in which the D-galacturonic acid is first oxidized to meso-galactaric acid .
Pharmacokinetics
It is known that the proportion of methylated galacturonic acid decreases during pectin extraction . More research is needed to fully understand the ADME properties of DL-Galacturonic acid and their impact on bioavailability.
Result of Action
The result of DL-Galacturonic acid’s action is the formation of pectin, a natural polymer mainly made from D-galacturonic acid monomers . Pectin is used as a gelling or filling agent in foods such as jellies, jams, desserts, and candies, and as a stabilizer in juices and milk-based drinks .
Action Environment
The action of DL-Galacturonic acid is influenced by environmental factors. For instance, in the absence of bivalent cations, pectin is water-soluble; in their presence, it forms a gel . Pectin can also form a gel at acidic pH in the presence of high concentrations of sugar .
Biochemical Analysis
Biochemical Properties
DL-Galacturonic acid is involved in several biochemical reactions, primarily in the catabolism of pectin. It interacts with various enzymes, proteins, and other biomolecules. One of the key enzymes involved is D-galacturonate reductase, which reduces DL-Galacturonic acid to L-galactonate. This reaction is crucial for the subsequent steps in the metabolic pathway that leads to the production of pyruvate and D-glyceraldehyde-3-phosphate .
Additionally, DL-Galacturonic acid interacts with uronate dehydrogenase, which oxidizes it to D-galactaric acid. This interaction is essential for the conversion of pectin into useful biochemicals . The compound also binds to specific transport proteins, such as the D-GalA transporter GAT-1, which facilitates its uptake into cells .
Cellular Effects
DL-Galacturonic acid influences various cellular processes. It has been shown to modulate intestinal immune activity and improve intestinal mucosal permeability in animal models . This compound also affects cell signaling pathways, particularly those involved in inflammation. For example, it can downregulate the Toll-like receptor-nuclear factor kappa B (TLR/NF-κB) pathway, reducing inflammation in the intestinal mucosa .
In addition, DL-Galacturonic acid impacts gene expression related to cell adhesion and cell wall integrity. It enhances the crosslinking of extensin, a cell wall structural protein, thereby restoring cell adhesion in defective mutants .
Molecular Mechanism
At the molecular level, DL-Galacturonic acid exerts its effects through various binding interactions with biomolecules. It binds to D-galacturonate reductase and uronate dehydrogenase, facilitating the reduction and oxidation reactions mentioned earlier . These interactions are crucial for the compound’s role in pectin catabolism.
Furthermore, DL-Galacturonic acid can inhibit or activate specific enzymes, depending on the context. For instance, it has been shown to inhibit certain enzymes involved in the degradation of pectin, thereby regulating the overall metabolic flux . Additionally, the compound can influence gene expression by interacting with transcription factors such as BcGaaR, which regulates the utilization of DL-Galacturonic acid in fungi .
Temporal Effects in Laboratory Settings
In laboratory settings, the effects of DL-Galacturonic acid can change over time. The compound’s stability and degradation are important factors to consider. Studies have shown that DL-Galacturonic acid is relatively stable under standard laboratory conditions, but its degradation can be accelerated under alkaline conditions . Long-term exposure to DL-Galacturonic acid can lead to changes in cellular function, particularly in the context of pectin metabolism .
Dosage Effects in Animal Models
The effects of DL-Galacturonic acid vary with different dosages in animal models. At low doses, the compound has been shown to improve intestinal mucosal permeability and reduce inflammation . At higher doses, DL-Galacturonic acid can have toxic effects, including disruptions in cellular metabolism and increased oxidative stress . These threshold effects highlight the importance of dosage optimization in therapeutic applications.
Metabolic Pathways
DL-Galacturonic acid is involved in several metabolic pathways, particularly those related to pectin catabolism. It is converted to L-galactonate by D-galacturonate reductase, and subsequently to pyruvate and D-glyceraldehyde-3-phosphate through a series of enzymatic reactions . These pathways are essential for the complete utilization of pectin-rich substrates in biotechnological applications .
Transport and Distribution
Within cells, DL-Galacturonic acid is transported and distributed by specific transport proteins. The D-GalA transporter GAT-1, identified in Neurospora crassa, plays a crucial role in the uptake of DL-Galacturonic acid into cells . This transporter ensures that the compound is efficiently absorbed and utilized in metabolic processes.
Subcellular Localization
The subcellular localization of DL-Galacturonic acid is primarily within the cytoplasm, where it participates in various metabolic reactions. Additionally, the compound can be found in the nucleus, particularly in the presence of specific transcription factors such as BcGaaR . This localization is essential for the regulation of gene expression related to pectin catabolism.
properties
{ "Design of the Synthesis Pathway": "The synthesis pathway for '(2S,3R,4S,5R)-2,3,4,5-tetrahydroxy-6-oxohexanoic acid' involves the conversion of a suitable starting material to the target compound through a series of chemical reactions.", "Starting Materials": [ "D-glucose", "Hydrogen peroxide", "Sodium hydroxide", "Sodium borohydride", "Sodium periodate", "Sodium chlorite", "Sodium bicarbonate", "Water" ], "Reaction": [ "Step 1: Oxidation of D-glucose with sodium periodate to form D-gluconic acid.", "Step 2: Reduction of D-gluconic acid with sodium borohydride to form D-gluconolactone.", "Step 3: Oxidation of D-gluconolactone with sodium chlorite and sodium bicarbonate to form (2S,3R,4S,5R)-2,3,4,5-tetrahydroxyhexanoic acid.", "Step 4: Conversion of (2S,3R,4S,5R)-2,3,4,5-tetrahydroxyhexanoic acid to (2S,3R,4S,5R)-2,3,4,5-tetrahydroxy-6-oxohexanoic acid by oxidation with hydrogen peroxide in the presence of sodium hydroxide." ] } | |
CAS RN |
685-73-4 |
Molecular Formula |
C6H10O7 |
Molecular Weight |
194.14 g/mol |
IUPAC Name |
2,3,4,5-tetrahydroxy-6-oxohexanoic acid |
InChI |
InChI=1S/C6H10O7/c7-1-2(8)3(9)4(10)5(11)6(12)13/h1-5,8-11H,(H,12,13) |
InChI Key |
IAJILQKETJEXLJ-UHFFFAOYSA-N |
Isomeric SMILES |
C(=O)[C@@H]([C@H]([C@H]([C@@H](C(=O)O)O)O)O)O |
SMILES |
C(=O)C(C(C(C(C(=O)O)O)O)O)O |
Canonical SMILES |
C(=O)C(C(C(C(C(=O)O)O)O)O)O |
melting_point |
165.5 °C |
Other CAS RN |
14982-50-4 34150-36-2 685-73-4 |
physical_description |
Solid |
Pictograms |
Irritant |
synonyms |
(D)-galacturonic acid (DL)-galacturonic acid D-galacturonic acid DL-galacturonic acid galacturonic acid galacturonic acid, (alpha-D)-isomer galacturonic acid, (D)-isomer galacturonic acid, calcium, sodium salt, (D)-isomer galacturonic acid, D- galacturonic acid, DL- galacturonic acid, monosodium salt, (D)-isome |
Origin of Product |
United States |
Retrosynthesis Analysis
AI-Powered Synthesis Planning: Our tool employs the Template_relevance Pistachio, Template_relevance Bkms_metabolic, Template_relevance Pistachio_ringbreaker, Template_relevance Reaxys, Template_relevance Reaxys_biocatalysis model, leveraging a vast database of chemical reactions to predict feasible synthetic routes.
One-Step Synthesis Focus: Specifically designed for one-step synthesis, it provides concise and direct routes for your target compounds, streamlining the synthesis process.
Accurate Predictions: Utilizing the extensive PISTACHIO, BKMS_METABOLIC, PISTACHIO_RINGBREAKER, REAXYS, REAXYS_BIOCATALYSIS database, our tool offers high-accuracy predictions, reflecting the latest in chemical research and data.
Strategy Settings
Precursor scoring | Relevance Heuristic |
---|---|
Min. plausibility | 0.01 |
Model | Template_relevance |
Template Set | Pistachio/Bkms_metabolic/Pistachio_ringbreaker/Reaxys/Reaxys_biocatalysis |
Top-N result to add to graph | 6 |
Feasible Synthetic Routes
Disclaimer and Information on In-Vitro Research Products
Please be aware that all articles and product information presented on BenchChem are intended solely for informational purposes. The products available for purchase on BenchChem are specifically designed for in-vitro studies, which are conducted outside of living organisms. In-vitro studies, derived from the Latin term "in glass," involve experiments performed in controlled laboratory settings using cells or tissues. It is important to note that these products are not categorized as medicines or drugs, and they have not received approval from the FDA for the prevention, treatment, or cure of any medical condition, ailment, or disease. We must emphasize that any form of bodily introduction of these products into humans or animals is strictly prohibited by law. It is essential to adhere to these guidelines to ensure compliance with legal and ethical standards in research and experimentation.