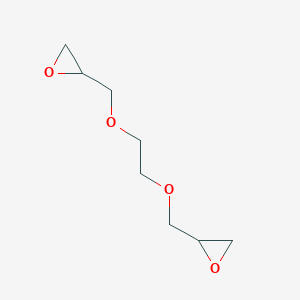
Ethylene glycol diglycidyl ether
Overview
Description
Ethylene glycol diglycidyl ether (EGDGE) is a chemical compound that is widely used in many industries, including the medical, automotive, and aerospace industries. EGDGE is a member of the epoxy family of compounds and is used primarily as a curing agent, adhesion promoter, and cross-linking agent. It is also used as a plasticizer and flame retardant. EGDGE is a colorless, odorless, and non-volatile liquid with a low viscosity. It is highly soluble in water and ethanol and is also highly reactive with other chemicals.
Scientific Research Applications
Synthesis of Oligo Ethylene Glycol Diglycidyl Ether : EGDE can be prepared from oligo ethylene glycol and epichlorohydrin via phase transfer catalysis with high selectivity for diether (98%) (Wu, 2005).
UV-Cured Coatings : EGDE can be synthesized under optimal conditions to enhance mechanical properties like tensile strength, Young's modulus, and elongation at tear for UV-cured coatings (Chen, 2011).
Polymer Electrolytes for Lithium-Ion Batteries : Gelled polymer electrolytes based on poly(ethylene glycol) diglycidyl ether show good mechanical properties and ionic conductivity, useful in lithium-ion batteries (Liang, Chen, & Kuo, 2004).
Protein Cross-Linking : EGDE can cross-link hemoglobin and other proteins effectively, without significantly impairing biological activity, offering an alternative to glutaraldehyde (Lu et al., 2006).
CO2 Separation Membranes : PEG-based membranes using EGDE for CO2 separation demonstrate excellent separation efficiency (Dai et al., 2017).
Ceramic Gel Casting : EGDE is effective for gel casting ceramics, providing suitable properties for this application (Mao et al., 2008).
Biomedical Nanocarriers : EGDE-functional poly(ethylene glycol) copolymers show promise as redox-responsive nanocarriers in biomedical applications (Herzberger et al., 2016).
Dye Removal : EGDE can enhance the efficiency of composites for removing dyes, with optimal conditions for fly ash loading into chitosan-ethylene glycol diglycidyl ether composites determined (Jawad, Mohammed, & Abdulhameed, 2020).
Wood Adhesive Enhancement : EGDE enhances soybean meal-based wood adhesive performance, improving wet shear strength and water resistance (Li et al., 2015).
Cross-Linked Hemoglobin : EGDE is used in preparing cross-linked hemoglobin as a potential red blood cell substitute (Xu et al., 2006).
Solid Polymer Electrolytes : EGDE contributes to the development of high-performance solid polymer electrolytes for rechargeable batteries (Cui et al., 2017).
Epoxy-Amine Systems : EGDE plays a role in the polymerization kinetics of modified epoxy-amine systems (Swier et al., 2005).
Blood Compatibility : EGDE crosslinked silk fibroin exhibits distinct anticoagulant characteristics in various coagulation pathways (Hao et al., 2015).
Aerospace Applications : Modified graphene nanoplatelet and epoxy/block copolymer-based nanocomposites with EGDE enhance physical properties and EMI shielding, suitable for aerospace applications (Anwar et al., 2016).
Biomedical Bioengineering : EGDE crosslinked PEDOT:PSS films improve conductivity and biocompatibility for bioengineering applications (Solazzo et al., 2019).
Toxicity Assessment : EGDE, particularly in higher concentrations, shows subcutaneous toxicity, indicating an optimal concentration for specific applications (Kim et al., 2021).
Ion-Conductive Polymers : EGDE-based networked polymers with quaternary ammonium structure exhibit high thermal stability and good ionic conductivity (Matsumoto & Endo, 2009).
Protein Separations : Cross-linked macroporous chitosan membranes with EGDE show potential for protein separations in ion-exchange mode (Zeng & Ruckenstein, 1998).
Multifunctional Biotechnological Applications : Plasmid DNA nanogels with unique properties have potential in gene and drug delivery applications (Costa, Valente, & Queiroz, 2015).
Biosensor Development : EGDE-immobilized enzymes on microelectrode biosensors offer a simple, low-cost, and non-toxic option for in vivo biological process monitoring (Vasylieva et al., 2011).
Mechanism of Action
Target of Action
Ethylene glycol diglycidyl ether (EGDE) is a common crosslinking agent due to its epoxy groups at both ends . These groups can react with various active groups such as amino, hydroxyl, and carboxyl . The primary targets of EGDE are therefore these active groups present in various molecules, particularly in proteins like soy protein isolate (SPI) .
Mode of Action
EGDE interacts with its targets through a ring-opening reaction with the epoxy groups . This interaction results in the formation of a dense three-dimensional network structure . For instance, when EGDE is used with SPI, it enhances the hydrogen bonding between SPI chains and other components, making the network structure of the resulting material denser .
Biochemical Pathways
The primary biochemical pathway affected by EGDE involves the crosslinking of polymers. In the case of SPI, EGDE and ultrasonic-modified montmorillonite (UMMT) have a synergistic effect on SPI molecules, enhancing the network structure of the resulting composite film . This modification strategy is considered effective for improving the functional properties of bio-based polymer composites .
Pharmacokinetics
Information on the ADME (Absorption, Distribution, Metabolism, and Excretion) properties of EGDE is limited. It’s known that egde is extremely toxic and can prove fatal or cause permanent damage if inhaled or consumed orally . Therefore, its bioavailability and impact on biological systems should be considered with caution.
Result of Action
The action of EGDE results in enhanced mechanical and barrier properties of the materials it is used with. For example, when used with SPI and UMMT to produce a protein-based composite film, the tensile strength of the film increased by 266.82% compared to a pure soy protein film . This demonstrates the significant impact of EGDE on the physical properties of the materials it interacts with.
Action Environment
The action of EGDE can be influenced by environmental factors. For instance, the dimensional stability and flexibility of materials crosslinked with EGDE can be affected by alternating dry-wet environmental conditions . Moreover, EGDE forms explosive mixtures with air on intense heating, and the development of hazardous combustion gases or vapors is possible in the event of fire .
Safety and Hazards
Biochemical Analysis
Biochemical Properties
Ethylene glycol diglycidyl ether plays a significant role in biochemical reactions as a crosslinking agent. It interacts with enzymes, proteins, and other biomolecules through its epoxy groups, which can react with amino, hydroxyl, carboxyl, and other active groups. For example, it can form covalent bonds with amino groups in proteins, leading to the formation of a dense three-dimensional network structure . This interaction enhances the mechanical and barrier properties of protein-based materials, such as soy protein isolate films . Additionally, this compound is used to immobilize enzymes, such as lipase, on carriers like sodium alginate and carboxymethyl cellulose sodium, improving their stability and activity .
Cellular Effects
This compound has various effects on different types of cells and cellular processes. It can influence cell function by modifying the extracellular matrix and altering cell signaling pathways. For instance, it has been shown to cause allergic skin reactions and irritation upon contact . In laboratory settings, this compound has been used to crosslink chitosan cryogels, which are then used for cell culturing applications . These cryogels provide a suitable environment for cell growth and proliferation, demonstrating the compound’s potential in tissue engineering and regenerative medicine.
Molecular Mechanism
The molecular mechanism of this compound involves its ability to form covalent bonds with various biomolecules through its epoxy groups. This crosslinking process can lead to enzyme inhibition or activation, depending on the specific interactions. For example, this compound can react with the amino groups of proteins, forming stable crosslinked structures that enhance the mechanical properties of the resulting materials . Additionally, it can modify the activity of enzymes by altering their conformation and stability .
Temporal Effects in Laboratory Settings
In laboratory settings, the effects of this compound can change over time due to its stability and degradation properties. The compound is relatively stable under normal conditions, but its reactivity can decrease over time as the epoxy groups are consumed in crosslinking reactions . Long-term studies have shown that this compound-crosslinked materials, such as chitosan cryogels, maintain their mechanical properties and biocompatibility over extended periods . The degradation rate of these materials can vary depending on the crosslinking conditions and the presence of enzymes that can hydrolyze the crosslinked structures .
Dosage Effects in Animal Models
The effects of this compound vary with different dosages in animal models. Studies have shown that high doses of the compound can cause toxic effects, such as epidermal ulcer formation and hair loss in mice . For example, subcutaneous injection of 20,000 micrograms of this compound in mice resulted in significant skin irritation and wound formation . Lower doses did not cause any adverse effects on the liver, spleen, or kidneys, indicating that the compound’s toxicity is dose-dependent .
Metabolic Pathways
This compound is not a naturally occurring metabolite and is only found in individuals exposed to the compound or its derivatives . It is part of the human exposome and can be metabolized through various pathways. For instance, it can be hydrolyzed by enzymes such as epoxide hydrolases, leading to the formation of less reactive diols . These metabolic pathways help to detoxify the compound and reduce its potential harmful effects on the body .
Transport and Distribution
The transport and distribution of this compound within cells and tissues are influenced by its chemical properties. The compound can diffuse through cell membranes and interact with intracellular biomolecules . Additionally, it can be transported by specific binding proteins or transporters that recognize its chemical structure . The localization and accumulation of this compound within cells can affect its reactivity and the extent of its interactions with biomolecules .
Subcellular Localization
This compound can localize to various subcellular compartments, depending on its interactions with targeting signals and post-translational modifications. For example, it can be directed to specific organelles, such as the endoplasmic reticulum or the Golgi apparatus, where it can participate in protein modification and crosslinking reactions . The subcellular localization of this compound can influence its activity and function, as well as its potential effects on cellular processes .
Properties
IUPAC Name |
2-[2-(oxiran-2-ylmethoxy)ethoxymethyl]oxirane | |
---|---|---|
Source | PubChem | |
URL | https://pubchem.ncbi.nlm.nih.gov | |
Description | Data deposited in or computed by PubChem | |
InChI |
InChI=1S/C8H14O4/c1(9-3-7-5-11-7)2-10-4-8-6-12-8/h7-8H,1-6H2 | |
Source | PubChem | |
URL | https://pubchem.ncbi.nlm.nih.gov | |
Description | Data deposited in or computed by PubChem | |
InChI Key |
AOBIOSPNXBMOAT-UHFFFAOYSA-N | |
Source | PubChem | |
URL | https://pubchem.ncbi.nlm.nih.gov | |
Description | Data deposited in or computed by PubChem | |
Canonical SMILES |
C1C(O1)COCCOCC2CO2 | |
Source | PubChem | |
URL | https://pubchem.ncbi.nlm.nih.gov | |
Description | Data deposited in or computed by PubChem | |
Molecular Formula |
C8H14O4 | |
Source | PubChem | |
URL | https://pubchem.ncbi.nlm.nih.gov | |
Description | Data deposited in or computed by PubChem | |
Related CAS |
26403-72-5, 58782-18-6, 29317-04-2 | |
Record name | Polyethylene glycol diglycidyl ether | |
Source | CAS Common Chemistry | |
URL | https://commonchemistry.cas.org/detail?cas_rn=26403-72-5 | |
Description | CAS Common Chemistry is an open community resource for accessing chemical information. Nearly 500,000 chemical substances from CAS REGISTRY cover areas of community interest, including common and frequently regulated chemicals, and those relevant to high school and undergraduate chemistry classes. This chemical information, curated by our expert scientists, is provided in alignment with our mission as a division of the American Chemical Society. | |
Explanation | The data from CAS Common Chemistry is provided under a CC-BY-NC 4.0 license, unless otherwise stated. | |
Record name | Poly(oxy-1,2-ethanediyl), α-(2-oxiranylmethyl)-ω-(2-oxiranylmethoxy)-, homopolymer | |
Source | CAS Common Chemistry | |
URL | https://commonchemistry.cas.org/detail?cas_rn=58782-18-6 | |
Description | CAS Common Chemistry is an open community resource for accessing chemical information. Nearly 500,000 chemical substances from CAS REGISTRY cover areas of community interest, including common and frequently regulated chemicals, and those relevant to high school and undergraduate chemistry classes. This chemical information, curated by our expert scientists, is provided in alignment with our mission as a division of the American Chemical Society. | |
Explanation | The data from CAS Common Chemistry is provided under a CC-BY-NC 4.0 license, unless otherwise stated. | |
Record name | Oxirane, 2,2′-[1,2-ethanediylbis(oxymethylene)]bis-, homopolymer | |
Source | CAS Common Chemistry | |
URL | https://commonchemistry.cas.org/detail?cas_rn=29317-04-2 | |
Description | CAS Common Chemistry is an open community resource for accessing chemical information. Nearly 500,000 chemical substances from CAS REGISTRY cover areas of community interest, including common and frequently regulated chemicals, and those relevant to high school and undergraduate chemistry classes. This chemical information, curated by our expert scientists, is provided in alignment with our mission as a division of the American Chemical Society. | |
Explanation | The data from CAS Common Chemistry is provided under a CC-BY-NC 4.0 license, unless otherwise stated. | |
DSSTOX Substance ID |
DTXSID4044876 | |
Record name | Ethylene glycol diglycidyl ether | |
Source | EPA DSSTox | |
URL | https://comptox.epa.gov/dashboard/DTXSID4044876 | |
Description | DSSTox provides a high quality public chemistry resource for supporting improved predictive toxicology. | |
Molecular Weight |
174.19 g/mol | |
Source | PubChem | |
URL | https://pubchem.ncbi.nlm.nih.gov | |
Description | Data deposited in or computed by PubChem | |
Physical Description |
Liquid | |
Record name | Oxirane, 2,2'-[1,2-ethanediylbis(oxymethylene)]bis- | |
Source | EPA Chemicals under the TSCA | |
URL | https://www.epa.gov/chemicals-under-tsca | |
Description | EPA Chemicals under the Toxic Substances Control Act (TSCA) collection contains information on chemicals and their regulations under TSCA, including non-confidential content from the TSCA Chemical Substance Inventory and Chemical Data Reporting. | |
CAS No. |
2224-15-9, 72207-80-8 | |
Record name | Glycol diglycidyl ether | |
Source | CAS Common Chemistry | |
URL | https://commonchemistry.cas.org/detail?cas_rn=2224-15-9 | |
Description | CAS Common Chemistry is an open community resource for accessing chemical information. Nearly 500,000 chemical substances from CAS REGISTRY cover areas of community interest, including common and frequently regulated chemicals, and those relevant to high school and undergraduate chemistry classes. This chemical information, curated by our expert scientists, is provided in alignment with our mission as a division of the American Chemical Society. | |
Explanation | The data from CAS Common Chemistry is provided under a CC-BY-NC 4.0 license, unless otherwise stated. | |
Record name | Bis(1,2-epoxypropylether)ethanediol | |
Source | ChemIDplus | |
URL | https://pubchem.ncbi.nlm.nih.gov/substance/?source=chemidplus&sourceid=0002224159 | |
Description | ChemIDplus is a free, web search system that provides access to the structure and nomenclature authority files used for the identification of chemical substances cited in National Library of Medicine (NLM) databases, including the TOXNET system. | |
Record name | Ethylene glycol diglycidyl ether | |
Source | DTP/NCI | |
URL | https://dtp.cancer.gov/dtpstandard/servlet/dwindex?searchtype=NSC&outputformat=html&searchlist=54740 | |
Description | The NCI Development Therapeutics Program (DTP) provides services and resources to the academic and private-sector research communities worldwide to facilitate the discovery and development of new cancer therapeutic agents. | |
Explanation | Unless otherwise indicated, all text within NCI products is free of copyright and may be reused without our permission. Credit the National Cancer Institute as the source. | |
Record name | Oxirane, 2,2'-[1,2-ethanediylbis(oxymethylene)]bis- | |
Source | EPA Chemicals under the TSCA | |
URL | https://www.epa.gov/chemicals-under-tsca | |
Description | EPA Chemicals under the Toxic Substances Control Act (TSCA) collection contains information on chemicals and their regulations under TSCA, including non-confidential content from the TSCA Chemical Substance Inventory and Chemical Data Reporting. | |
Record name | Ethylene glycol diglycidyl ether | |
Source | EPA DSSTox | |
URL | https://comptox.epa.gov/dashboard/DTXSID4044876 | |
Description | DSSTox provides a high quality public chemistry resource for supporting improved predictive toxicology. | |
Record name | 2,2'-[ethylenebis(oxymethylene)]bisoxirane | |
Source | European Chemicals Agency (ECHA) | |
URL | https://echa.europa.eu/substance-information/-/substanceinfo/100.017.043 | |
Description | The European Chemicals Agency (ECHA) is an agency of the European Union which is the driving force among regulatory authorities in implementing the EU's groundbreaking chemicals legislation for the benefit of human health and the environment as well as for innovation and competitiveness. | |
Explanation | Use of the information, documents and data from the ECHA website is subject to the terms and conditions of this Legal Notice, and subject to other binding limitations provided for under applicable law, the information, documents and data made available on the ECHA website may be reproduced, distributed and/or used, totally or in part, for non-commercial purposes provided that ECHA is acknowledged as the source: "Source: European Chemicals Agency, http://echa.europa.eu/". Such acknowledgement must be included in each copy of the material. ECHA permits and encourages organisations and individuals to create links to the ECHA website under the following cumulative conditions: Links can only be made to webpages that provide a link to the Legal Notice page. | |
Record name | Polyethylene glycol diglycidyl ether | |
Source | European Chemicals Agency (ECHA) | |
URL | https://echa.europa.eu/information-on-chemicals | |
Description | The European Chemicals Agency (ECHA) is an agency of the European Union which is the driving force among regulatory authorities in implementing the EU's groundbreaking chemicals legislation for the benefit of human health and the environment as well as for innovation and competitiveness. | |
Explanation | Use of the information, documents and data from the ECHA website is subject to the terms and conditions of this Legal Notice, and subject to other binding limitations provided for under applicable law, the information, documents and data made available on the ECHA website may be reproduced, distributed and/or used, totally or in part, for non-commercial purposes provided that ECHA is acknowledged as the source: "Source: European Chemicals Agency, http://echa.europa.eu/". Such acknowledgement must be included in each copy of the material. ECHA permits and encourages organisations and individuals to create links to the ECHA website under the following cumulative conditions: Links can only be made to webpages that provide a link to the Legal Notice page. | |
Record name | ETHYLENE GLYCOL DIGLYCIDYL ETHER | |
Source | FDA Global Substance Registration System (GSRS) | |
URL | https://gsrs.ncats.nih.gov/ginas/app/beta/substances/5R860RS597 | |
Description | The FDA Global Substance Registration System (GSRS) enables the efficient and accurate exchange of information on what substances are in regulated products. Instead of relying on names, which vary across regulatory domains, countries, and regions, the GSRS knowledge base makes it possible for substances to be defined by standardized, scientific descriptions. | |
Explanation | Unless otherwise noted, the contents of the FDA website (www.fda.gov), both text and graphics, are not copyrighted. They are in the public domain and may be republished, reprinted and otherwise used freely by anyone without the need to obtain permission from FDA. Credit to the U.S. Food and Drug Administration as the source is appreciated but not required. | |
Synthesis routes and methods
Procedure details
Retrosynthesis Analysis
AI-Powered Synthesis Planning: Our tool employs the Template_relevance Pistachio, Template_relevance Bkms_metabolic, Template_relevance Pistachio_ringbreaker, Template_relevance Reaxys, Template_relevance Reaxys_biocatalysis model, leveraging a vast database of chemical reactions to predict feasible synthetic routes.
One-Step Synthesis Focus: Specifically designed for one-step synthesis, it provides concise and direct routes for your target compounds, streamlining the synthesis process.
Accurate Predictions: Utilizing the extensive PISTACHIO, BKMS_METABOLIC, PISTACHIO_RINGBREAKER, REAXYS, REAXYS_BIOCATALYSIS database, our tool offers high-accuracy predictions, reflecting the latest in chemical research and data.
Strategy Settings
Precursor scoring | Relevance Heuristic |
---|---|
Min. plausibility | 0.01 |
Model | Template_relevance |
Template Set | Pistachio/Bkms_metabolic/Pistachio_ringbreaker/Reaxys/Reaxys_biocatalysis |
Top-N result to add to graph | 6 |
Feasible Synthetic Routes
Q1: What is ethylene glycol diglycidyl ether primarily used for?
A1: this compound (EGDE) is primarily used as a crosslinking agent, a viscosity reducer, and a plasticizing agent. [, ]
Q2: How does EGDE function as a crosslinking agent?
A2: EGDE contains two epoxy groups that can react with various functional groups, such as amines, hydroxyls, and carboxyls, forming stable crosslinks between polymer chains. [, , ]
Q3: Can you give examples of materials where EGDE is used as a crosslinking agent?
A3: EGDE is used to crosslink a variety of materials, including soy protein-based adhesives, chitosan hydrogels, and poly(vinyl alcohol) films, enhancing their mechanical strength, thermal stability, and water resistance. [, , , , ]
Q4: How does EGDE impact the properties of soy-based adhesives?
A4: Adding EGDE to soy-based adhesives can significantly reduce viscosity, increase solid content, and improve wet shear strength by facilitating crosslinking between soy protein molecules and other components like polyvinyl alcohol. []
Q5: EGDE is used in gel-casting of ceramics. Why is it suitable for this application?
A5: this compound (EGDGE) exhibits excellent solubility in water and allows for controlled gelation, making it suitable for the gel casting of ceramics in an air atmosphere. []
Q6: Can EGDE be used with nanomaterials to further enhance material properties?
A6: Yes, research shows that combining EGDE with ultrasonically modified montmorillonite can synergistically enhance the mechanical and barrier properties of soy protein isolate films. The modified montmorillonite interacts with soy protein chains, and EGDE further strengthens the network through crosslinking. []
Q7: What are the biomedical applications of EGDE?
A7: EGDE is being investigated for applications in drug delivery systems and tissue engineering due to its ability to form hydrogels and crosslink biopolymers. [, , , , , ]
Q8: How is EGDE used in drug delivery systems?
A8: EGDE can be used to create crosslinked chitosan hydrogels that encapsulate and control the release of drugs like amoxicillin. The release can be further modulated by external stimuli like ultrasound. []
Q9: Are there specific tissue engineering applications where EGDE shows promise?
A9: Research indicates EGDE-crosslinked silk fibroin hydrogels could be beneficial in wound healing. These hydrogels, particularly when loaded with superoxide dismutase, have demonstrated accelerated wound closure, increased collagen deposition, and enhanced epithelialization in diabetic rat models. []
Q10: What challenges are associated with EGDE-treated bioprosthetic heart valves?
A10: While EGDE treatment improves the durability of bioprosthetic heart valves, a major concern is their susceptibility to calcification, leading to structural valve degeneration. Studies are exploring the mechanisms of this calcification to develop strategies for its prevention. [, ]
Q11: How does pH influence the reaction of EGDE with proteins like hemoglobin?
A11: EGDE exhibits pH-dependent reactivity with proteins. At a neutral pH of 7.5, it reacts preferentially with sulfhydryl groups, while at a higher pH of 9.5, the reaction predominantly occurs with amino groups. [, ]
Q12: Can you elaborate on the use of EGDE in hemoglobin crosslinking?
A12: EGDE is investigated as a potential alternative to glutaraldehyde for crosslinking hemoglobin to develop hemoglobin-based oxygen carriers (HBOCs). By controlling the reaction conditions, researchers can produce both intra- and inter-molecularly crosslinked hemoglobin with desired oxygen-carrying properties. [, ]
Q13: What are some key areas for future research on EGDE?
A13: Future research on EGDE should focus on: * Fully characterizing its long-term safety and biocompatibility.* Optimizing its use in drug delivery systems for improved targeting and controlled release.* Exploring its potential in developing new biomaterials with enhanced properties.* Investigating its environmental fate and developing sustainable practices for its use and disposal.
Q14: What are the potential advantages of EGDE over other crosslinking agents?
A14: EGDE offers advantages such as low viscosity, good solubility in water and other solvents, and the ability to tailor its reactivity by adjusting pH. These properties make it a versatile crosslinking agent for various applications. [, , ]
Disclaimer and Information on In-Vitro Research Products
Please be aware that all articles and product information presented on BenchChem are intended solely for informational purposes. The products available for purchase on BenchChem are specifically designed for in-vitro studies, which are conducted outside of living organisms. In-vitro studies, derived from the Latin term "in glass," involve experiments performed in controlled laboratory settings using cells or tissues. It is important to note that these products are not categorized as medicines or drugs, and they have not received approval from the FDA for the prevention, treatment, or cure of any medical condition, ailment, or disease. We must emphasize that any form of bodily introduction of these products into humans or animals is strictly prohibited by law. It is essential to adhere to these guidelines to ensure compliance with legal and ethical standards in research and experimentation.