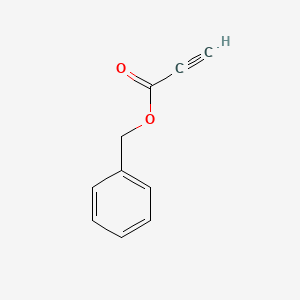
Benzyl propiolate
Overview
Description
Synthesis Analysis
The synthesis of benzyl propiolate and its derivatives involves several innovative methods. For instance, benzyl esters of propiolic and beta-keto acids can undergo catalytic decarboxylative coupling using palladium catalysts, facilitating the benzylation of alkynes without the need for strong bases or organometallics, enabling the synthesis of sensitive benzylic alkynes (Torregrosa et al., 2010). Moreover, copper-promoted coupling with terminal alkynes, including electron-poor acetylenes like methyl propiolate, allows for easy access to a range of benzyl-substituted propiolates, showcasing its versatility as a building block for synthesizing heterocycles (Davies et al., 2009).
Molecular Structure Analysis
The molecular structure and characterization of this compound derivatives have been explored through various spectroscopic methods. In a study on the synthesis and structural investigation of a symmetrically trisubstituted benzene derivative, spectroscopic analysis including NMR and FTIR measurements was performed, revealing the structural intricacies of these compounds (Münch et al., 2013).
Chemical Reactions and Properties
This compound participates in diverse chemical reactions, leading to the formation of various complex molecules. For instance, gold-catalyzed reactions between 1,2-benzisoxazoles and propiolate derivatives exhibit ester-controlled chemoselectivity, yielding Michael-type products or [4 + 2]-annulations to form 6H-1,3-oxazin-6-one derivatives, demonstrating the compound's reactivity and versatility (Pandit et al., 2018).
Physical Properties Analysis
The physical properties of this compound derivatives, such as melting points, boiling points, and solubility, are crucial for their application in synthetic chemistry. Although the search did not return specific studies focusing solely on the physical properties of this compound, these properties are typically inferred from the molecular structure and are essential for determining the conditions under which these compounds can be synthesized and used in reactions.
Chemical Properties Analysis
The chemical properties of this compound, including reactivity, stability, and compatibility with various functional groups, are fundamental to its application in organic synthesis. The compound's ability to undergo decarboxylative coupling and participate in Michael-type reactions and [4 + 2]-annulations underscores its chemical versatility (Pandit et al., 2018; Torregrosa et al., 2010).
Scientific Research Applications
Synthesis of Heterocycles and Alkynes Benzyl propiolate shows significant potential in the synthesis of functionalized heterocycles and related alkynes. Davies, Abel, and Wulff (2009) demonstrated that benzyl chlorides and bromides could undergo copper-promoted coupling with various terminal alkynes, including electron-poor acetylenes like methyl propiolate. This process facilitates easy access to a range of benzyl-substituted propiolates, which are valuable for creating previously inaccessible benzyl-substituted heterocycles (Davies, Abel, & Wulff, 2009).
Catalytic Decarboxylative Coupling In 2010, Torregrosa et al. explored the catalytic decarboxylative coupling of benzyl esters of propiolic acids. This method allowed for the benzylation of alkynes without requiring strong bases or organometallics, enabling the synthesis of sensitive benzylic alkynes prone to isomerizations under basic conditions (Torregrosa et al., 2010).
Activation in Gold Nanoparticle Catalysis Research by Leyva–Pérez et al. (2013) demonstrated the cyclotrimerization of propiolates in the presence of catalytic amounts of gold nanoparticles, leading to the formation of aryl benzoates. This study highlighted how gold nanoparticles can modify the electronic density of unsaturated C–C bonds in propiolates, suggesting new avenues for gold-catalyzed transformations (Leyva–Pérez et al., 2013).
Synthesis of Diaryl 1,2-Diketones The work of Min et al. (2014) focused on synthesizing benzil derivatives, such as diaryl 1,2-diketones, through direct decarboxylative coupling of aryl propiolic acids. This method showed good functional group tolerance and provided an efficient way to synthesize symmetric diaryl 1,2-diketones using palladium and copper catalysts (Min et al., 2014).
Stereospecific Decarboxylative Benzylation Mendis and Tunge (2015) developed a stereospecific decarboxylative benzylation method using enantioenriched benzyl esters of propiolic acids. This sp-sp3 coupling did not require basic conditions and efficiently transferred stereochemical information from secondary benzyl alcohols to generate enantioenriched tertiary diarylmethanes (Mendis & Tunge, 2015).
Esterification for Perfumery and Flavor Industries Chandane et al. (2017) investigated the synthesis of benzyl propionate, an ester with floral and fruity odor used in perfumery and flavor industries. They optimized the synthesis process catalyzed by Amberlyst-15, demonstrating the absence of external and internal mass transfer limitations and validating the pseudohomogeneous (PH) kinetic model used (Chandane et al., 2017).
Potential in Lithium-Ion Batteries Renault et al. (2016) studied dilithium benzenedipropiolate as a potential negative electrode material for lithium-ion batteries. They found that this material could reduce and reversibly oxidize its unsaturated carbon–carbon bonds, leading to a high specific capacity. This research suggests new possibilities for using benzyl propiolates in energy storage technologies (Renault et al., 2016).
Safety and Hazards
Mechanism of Action
Target of Action
Benzyl propiolate, also known as benzyl propionate, is primarily used in the perfume and flavor industries . It’s important to note that the compound’s primary use is for its aromatic properties rather than a therapeutic effect.
Mode of Action
In the context of its use in perfumery and flavor industries, this compound interacts with olfactory receptors in the nose, leading to the perception of a sweet, fruity, and floral aroma .
Biochemical Pathways
It’s known that aromatic compounds like this compound can be synthesized from carboxylic acids and alcohols in a process known as esterification . In the case of this compound, this involves the reaction of propionic acid with benzyl alcohol .
Pharmacokinetics
Once absorbed, it may be metabolized by enzymes in the liver and excreted through the kidneys .
Result of Action
The primary result of this compound’s action is the perception of a sweet, fruity, and floral aroma when it interacts with olfactory receptors in the nose . This makes it a valuable ingredient in the creation of perfumes and flavorings.
Action Environment
The action of this compound can be influenced by various environmental factors. For instance, the compound’s volatility and therefore its ability to reach olfactory receptors can be affected by temperature and humidity. Additionally, the compound’s stability and efficacy can be influenced by factors such as pH and exposure to light or oxygen .
Biochemical Analysis
Biochemical Properties
Benzyl propiolate plays a significant role in biochemical reactions, particularly in the synthesis of complex organic molecules. It interacts with various enzymes, proteins, and other biomolecules. One notable interaction is with lipases, which catalyze the esterification process involving this compound. This interaction is crucial for the synthesis of aromatic esters, which are widely used in the food, cosmetic, and pharmaceutical industries . Additionally, this compound can act as a substrate for certain oxidoreductases, facilitating redox reactions that are essential for cellular metabolism.
Cellular Effects
This compound influences various cellular processes, including cell signaling pathways, gene expression, and cellular metabolism. It has been observed to affect the activity of key signaling molecules, such as kinases and phosphatases, which play a role in regulating cell growth and differentiation . Furthermore, this compound can modulate gene expression by interacting with transcription factors and other regulatory proteins. This modulation can lead to changes in the expression of genes involved in metabolic pathways, stress responses, and cell cycle regulation .
Molecular Mechanism
The molecular mechanism of this compound involves its binding interactions with biomolecules, enzyme inhibition or activation, and changes in gene expression. This compound can bind to active sites of enzymes, either inhibiting or activating their catalytic functions. For example, it can inhibit the activity of certain hydrolases by forming a stable complex with the enzyme, thereby preventing substrate binding . Additionally, this compound can activate transcription factors by inducing conformational changes that enhance their DNA-binding affinity, leading to altered gene expression patterns .
Temporal Effects in Laboratory Settings
In laboratory settings, the effects of this compound can change over time due to its stability and degradation. This compound is relatively stable under standard laboratory conditions, but it can undergo hydrolysis and oxidation over extended periods . These degradation processes can affect its efficacy in biochemical assays and long-term studies. In in vitro studies, this compound has been shown to maintain its activity for several hours, but its effects may diminish over time due to gradual degradation . In in vivo studies, the long-term effects of this compound on cellular function are still being investigated, with some studies indicating potential impacts on cellular homeostasis and metabolic regulation .
Dosage Effects in Animal Models
The effects of this compound vary with different dosages in animal models. At low doses, this compound has been observed to enhance metabolic activity and promote cellular growth . At high doses, it can exhibit toxic effects, including oxidative stress and cellular apoptosis . Threshold effects have been identified, where specific dosages result in significant changes in cellular responses. For instance, doses above a certain threshold can lead to the activation of stress response pathways and the induction of cell death . These findings highlight the importance of dosage optimization in experimental studies involving this compound.
Metabolic Pathways
This compound is involved in several metabolic pathways, including those related to fatty acid metabolism and redox reactions. It interacts with enzymes such as acyl-CoA synthetases and oxidoreductases, which facilitate its incorporation into metabolic processes . This compound can also influence metabolic flux by altering the levels of key metabolites, such as acetyl-CoA and NADH . These changes can impact overall cellular metabolism and energy production.
Transport and Distribution
Within cells and tissues, this compound is transported and distributed through various mechanisms. It can interact with transporters and binding proteins that facilitate its movement across cellular membranes . Once inside the cell, this compound can localize to specific compartments, such as the cytoplasm and mitochondria, where it exerts its biochemical effects . The distribution of this compound within tissues can also be influenced by factors such as blood flow and tissue permeability .
Subcellular Localization
The subcellular localization of this compound is critical for its activity and function. This compound can be targeted to specific organelles, such as the endoplasmic reticulum and mitochondria, through targeting signals and post-translational modifications . These localizations enable this compound to interact with organelle-specific enzymes and proteins, thereby influencing organelle function and cellular homeostasis . Understanding the subcellular localization of this compound is essential for elucidating its role in cellular processes and metabolic regulation.
properties
IUPAC Name |
benzyl prop-2-ynoate | |
---|---|---|
Source | PubChem | |
URL | https://pubchem.ncbi.nlm.nih.gov | |
Description | Data deposited in or computed by PubChem | |
InChI |
InChI=1S/C10H8O2/c1-2-10(11)12-8-9-6-4-3-5-7-9/h1,3-7H,8H2 | |
Source | PubChem | |
URL | https://pubchem.ncbi.nlm.nih.gov | |
Description | Data deposited in or computed by PubChem | |
InChI Key |
KCSLTHXLCZSZLB-UHFFFAOYSA-N | |
Source | PubChem | |
URL | https://pubchem.ncbi.nlm.nih.gov | |
Description | Data deposited in or computed by PubChem | |
Canonical SMILES |
C#CC(=O)OCC1=CC=CC=C1 | |
Source | PubChem | |
URL | https://pubchem.ncbi.nlm.nih.gov | |
Description | Data deposited in or computed by PubChem | |
Molecular Formula |
C10H8O2 | |
Source | PubChem | |
URL | https://pubchem.ncbi.nlm.nih.gov | |
Description | Data deposited in or computed by PubChem | |
DSSTOX Substance ID |
DTXSID80337487 | |
Record name | Benzyl propiolate | |
Source | EPA DSSTox | |
URL | https://comptox.epa.gov/dashboard/DTXSID80337487 | |
Description | DSSTox provides a high quality public chemistry resource for supporting improved predictive toxicology. | |
Molecular Weight |
160.17 g/mol | |
Source | PubChem | |
URL | https://pubchem.ncbi.nlm.nih.gov | |
Description | Data deposited in or computed by PubChem | |
CAS RN |
14447-01-9 | |
Record name | Benzyl propiolate | |
Source | EPA DSSTox | |
URL | https://comptox.epa.gov/dashboard/DTXSID80337487 | |
Description | DSSTox provides a high quality public chemistry resource for supporting improved predictive toxicology. | |
Record name | Benzyl propiolate | |
Source | European Chemicals Agency (ECHA) | |
URL | https://echa.europa.eu/information-on-chemicals | |
Description | The European Chemicals Agency (ECHA) is an agency of the European Union which is the driving force among regulatory authorities in implementing the EU's groundbreaking chemicals legislation for the benefit of human health and the environment as well as for innovation and competitiveness. | |
Explanation | Use of the information, documents and data from the ECHA website is subject to the terms and conditions of this Legal Notice, and subject to other binding limitations provided for under applicable law, the information, documents and data made available on the ECHA website may be reproduced, distributed and/or used, totally or in part, for non-commercial purposes provided that ECHA is acknowledged as the source: "Source: European Chemicals Agency, http://echa.europa.eu/". Such acknowledgement must be included in each copy of the material. ECHA permits and encourages organisations and individuals to create links to the ECHA website under the following cumulative conditions: Links can only be made to webpages that provide a link to the Legal Notice page. | |
Synthesis routes and methods I
Procedure details
Synthesis routes and methods II
Procedure details
Retrosynthesis Analysis
AI-Powered Synthesis Planning: Our tool employs the Template_relevance Pistachio, Template_relevance Bkms_metabolic, Template_relevance Pistachio_ringbreaker, Template_relevance Reaxys, Template_relevance Reaxys_biocatalysis model, leveraging a vast database of chemical reactions to predict feasible synthetic routes.
One-Step Synthesis Focus: Specifically designed for one-step synthesis, it provides concise and direct routes for your target compounds, streamlining the synthesis process.
Accurate Predictions: Utilizing the extensive PISTACHIO, BKMS_METABOLIC, PISTACHIO_RINGBREAKER, REAXYS, REAXYS_BIOCATALYSIS database, our tool offers high-accuracy predictions, reflecting the latest in chemical research and data.
Strategy Settings
Precursor scoring | Relevance Heuristic |
---|---|
Min. plausibility | 0.01 |
Model | Template_relevance |
Template Set | Pistachio/Bkms_metabolic/Pistachio_ringbreaker/Reaxys/Reaxys_biocatalysis |
Top-N result to add to graph | 6 |
Feasible Synthetic Routes
Q & A
Q1: What types of reactions can benzyl propiolate undergo, and how does its structure influence regioselectivity in these reactions?
A1: this compound is a versatile compound capable of participating in various reactions, including Diels-Alder reactions and 1,3-dipolar cycloadditions. [, ] The presence of the phenyl group in this compound can influence the regioselectivity of these reactions. For instance, in 1,3-dipolar cycloadditions with benzonitrile oxide, polymer-bound this compound yielded a single regioisomer upon cleavage and esterification. In contrast, the analogous reaction in solution produced a 1:1 mixture of two possible regioisomers. [] This highlights how attaching this compound to a polymer support can impact the reaction outcome compared to solution-phase chemistry.
Q2: Can you provide examples of how this compound has been utilized in green chemistry strategies?
A2: Researchers have explored the use of this compound in developing green chemistry approaches for synthesizing compounds with adjacent quaternary centers. [, ] Specifically, they employed a crystal-to-crystal photodecarbonylation reaction of a trans-α,α‘-dialkenoylcyclohexanone derivative synthesized from this compound. This solid-state reaction, using sunlight as an energy source, provided high yields of the desired product with excellent stereoselectivity, highlighting the potential of this compound in environmentally friendly synthetic strategies. [, ]
Q3: What is the molecular formula and weight of this compound?
A3: The molecular formula of this compound is C10H8O2, and its molecular weight is 160.17 g/mol.
Disclaimer and Information on In-Vitro Research Products
Please be aware that all articles and product information presented on BenchChem are intended solely for informational purposes. The products available for purchase on BenchChem are specifically designed for in-vitro studies, which are conducted outside of living organisms. In-vitro studies, derived from the Latin term "in glass," involve experiments performed in controlled laboratory settings using cells or tissues. It is important to note that these products are not categorized as medicines or drugs, and they have not received approval from the FDA for the prevention, treatment, or cure of any medical condition, ailment, or disease. We must emphasize that any form of bodily introduction of these products into humans or animals is strictly prohibited by law. It is essential to adhere to these guidelines to ensure compliance with legal and ethical standards in research and experimentation.