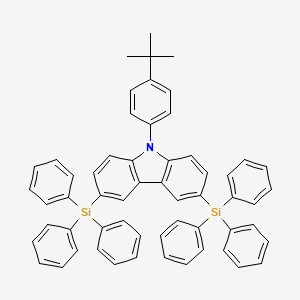
CzSi
Übersicht
Beschreibung
CzSi, also known as 9-(4-tert-Butylphenyl)-3,6-bis(triphenylsilyl)-9H-carbazole, is known for its high triplet energy, wide band-gap, and high glass-transition temperature (Tg >131 °C). It is one of the most widely-used TADF host materials for blue electrophosphorescence .
Synthesis Analysis
This compound is used in the creation of blue thermally activated delayed fluorescence organic light-emitting diodes . It is co-doped into a light-emitting layer (EML) as an electron transport ladder .Molecular Structure Analysis
This compound possesses bulky, sterically-hindered triphenylsilyl substitutions on electrochemically-active C3 and C6 positions of carbazole . It has a nearly-perfect 90° dihedral angle between its carbazole and tert-butylphenyl groups .Chemical Reactions Analysis
This compound is used in the formation of blue fluorescent electroluminescence by co-doping it into a light-emitting layer (EML) as an electron transport ladder . The performances of the co-doped device were improved effectively because of the well-matched energy levels and excellent electron transport ability of this compound .Physical and Chemical Properties Analysis
This compound has a high triplet energy, wide band-gap, and high glass-transition temperature . It exhibits enhanced morphological and superior electrochemical stability . It has a molecular weight of 816.19 g/mol, and its HOMO and LUMO values are 6.0 eV and 2.5 eV respectively .Wissenschaftliche Forschungsanwendungen
Czochralski-Silizium (CzSi) Anwendungen in der wissenschaftlichen Forschung
Halbleiterfertigung: Czochralski-Silizium ist in der Halbleiterindustrie von entscheidender Bedeutung aufgrund seiner hohen Reinheit und fehlerfreien Beschaffenheit, die für die Herstellung effizienter Halbleiterbauelemente unerlässlich ist. Die Kontrolle von Defekten und Verunreinigungen ist entscheidend, da diese die Leistung von Halbleiterbauelementen erheblich beeinträchtigen können .
Produktion von Mikro-/Computerchips: this compound-Wafer werden in großem Umfang bei der Herstellung von Mikrochips und Computerchips eingesetzt. Die Qualität von Siliziumwafern wirkt sich direkt auf die Leistung, Effizienz und Miniaturisierung dieser Chips aus, die für die moderne Datenverarbeitung von entscheidender Bedeutung sind .
Solarzellenherstellung: Hocheffiziente Solarzellen basieren oft auf this compound-Wafern. Das Wachstumsprozess zielt darauf ab, fehlerfreie Einkristalle zu erzeugen, die für fortschrittliche Solarzellenwafer aufgrund ihrer Auswirkungen auf die Leistung der Solarzelle unerlässlich sind .
Luft- und Raumfahrt: Die Luft- und Raumfahrtindustrie nutzt this compound-Wafer für verschiedene Anwendungen, darunter Satellitentechnologie, bei der Zuverlässigkeit und Effizienz von größter Bedeutung sind. Die Materialeigenschaften machen es für die rauen Bedingungen im Weltraum geeignet .
Elektronische Bauelemente & integrierte Schaltungen: this compound wird zur Herstellung elektronischer Bauelemente und integrierter Schaltungen verwendet. Seine elektrischen Eigenschaften sind unerlässlich für die Entwicklung von Geräten mit hoher Zuverlässigkeit und spezifischen Leistungseigenschaften .
Sensoren: Sensortechnologie integriert oft this compound aufgrund seiner stabilen elektrischen Eigenschaften, die für genaue Messungen und zuverlässigen Sensorenbetrieb in verschiedenen Anwendungen erforderlich sind .
Wirkmechanismus
Target of Action
The primary target of the compound 9-(4-tert-Butylphenyl)-3,6-bis(triphenylsilyl)-9H-carbazole, commonly known as CzSi, is in the field of optoelectronics . This compound is a Thermally Activated Delayed Fluorescence (TADF) host material used for blue electrophosphorescence . It is known for its high triplet energy, wide band-gap, and high glass-transition temperature .
Mode of Action
This compound interacts with its targets by exhibiting enhanced morphological and superior electrochemical stability . It possesses bulky, sterically-hindered triphenylsilyl substitutions on electrochemically-active C3 and C6 positions of carbazole . With a nearly-perfect 90° dihedral angle between its carbazole and tert-butylphenyl groups, this compound exhibits enhanced morphological and superior electrochemical stability .
Biochemical Pathways
The biochemical pathways affected by this compound are primarily related to the processes of electrophosphorescence. The compound’s high triplet energy and wide band-gap make it an ideal host material for blue electrophosphorescence . The downstream effects of these pathways include the production of blue light in organic light-emitting diodes .
Result of Action
The molecular and cellular effects of this compound’s action are most evident in its role in optoelectronics. When used as a host material in organic light-emitting diodes, this compound contributes to the production of blue light . This is a direct result of its interaction with other materials in the diode, leading to electrophosphorescence .
Action Environment
The action, efficacy, and stability of this compound can be influenced by various environmental factors. For instance, the compound’s high glass-transition temperature suggests that it can maintain stability under relatively high-temperature conditions . Additionally, its use in optoelectronics often requires a controlled environment to ensure optimal performance and longevity .
Eigenschaften
IUPAC Name |
[9-(4-tert-butylphenyl)-6-triphenylsilylcarbazol-3-yl]-triphenylsilane | |
---|---|---|
Source | PubChem | |
URL | https://pubchem.ncbi.nlm.nih.gov | |
Description | Data deposited in or computed by PubChem | |
InChI |
InChI=1S/C58H49NSi2/c1-58(2,3)44-34-36-45(37-35-44)59-56-40-38-52(60(46-22-10-4-11-23-46,47-24-12-5-13-25-47)48-26-14-6-15-27-48)42-54(56)55-43-53(39-41-57(55)59)61(49-28-16-7-17-29-49,50-30-18-8-19-31-50)51-32-20-9-21-33-51/h4-43H,1-3H3 | |
Source | PubChem | |
URL | https://pubchem.ncbi.nlm.nih.gov | |
Description | Data deposited in or computed by PubChem | |
InChI Key |
WIHKEPSYODOQJR-UHFFFAOYSA-N | |
Source | PubChem | |
URL | https://pubchem.ncbi.nlm.nih.gov | |
Description | Data deposited in or computed by PubChem | |
Canonical SMILES |
CC(C)(C)C1=CC=C(C=C1)N2C3=C(C=C(C=C3)[Si](C4=CC=CC=C4)(C5=CC=CC=C5)C6=CC=CC=C6)C7=C2C=CC(=C7)[Si](C8=CC=CC=C8)(C9=CC=CC=C9)C1=CC=CC=C1 | |
Source | PubChem | |
URL | https://pubchem.ncbi.nlm.nih.gov | |
Description | Data deposited in or computed by PubChem | |
Molecular Formula |
C58H49NSi2 | |
Source | PubChem | |
URL | https://pubchem.ncbi.nlm.nih.gov | |
Description | Data deposited in or computed by PubChem | |
DSSTOX Substance ID |
DTXSID80694860 | |
Record name | 9-(4-tert-Butylphenyl)-3,6-bis(triphenylsilyl)-9H-carbazole | |
Source | EPA DSSTox | |
URL | https://comptox.epa.gov/dashboard/DTXSID80694860 | |
Description | DSSTox provides a high quality public chemistry resource for supporting improved predictive toxicology. | |
Molecular Weight |
816.2 g/mol | |
Source | PubChem | |
URL | https://pubchem.ncbi.nlm.nih.gov | |
Description | Data deposited in or computed by PubChem | |
CAS No. |
898546-82-2 | |
Record name | 9-(4-tert-Butylphenyl)-3,6-bis(triphenylsilyl)-9H-carbazole | |
Source | EPA DSSTox | |
URL | https://comptox.epa.gov/dashboard/DTXSID80694860 | |
Description | DSSTox provides a high quality public chemistry resource for supporting improved predictive toxicology. | |
Retrosynthesis Analysis
AI-Powered Synthesis Planning: Our tool employs the Template_relevance Pistachio, Template_relevance Bkms_metabolic, Template_relevance Pistachio_ringbreaker, Template_relevance Reaxys, Template_relevance Reaxys_biocatalysis model, leveraging a vast database of chemical reactions to predict feasible synthetic routes.
One-Step Synthesis Focus: Specifically designed for one-step synthesis, it provides concise and direct routes for your target compounds, streamlining the synthesis process.
Accurate Predictions: Utilizing the extensive PISTACHIO, BKMS_METABOLIC, PISTACHIO_RINGBREAKER, REAXYS, REAXYS_BIOCATALYSIS database, our tool offers high-accuracy predictions, reflecting the latest in chemical research and data.
Strategy Settings
Precursor scoring | Relevance Heuristic |
---|---|
Min. plausibility | 0.01 |
Model | Template_relevance |
Template Set | Pistachio/Bkms_metabolic/Pistachio_ringbreaker/Reaxys/Reaxys_biocatalysis |
Top-N result to add to graph | 6 |
Feasible Synthetic Routes
Q1: Can the heat zone design in a CZSi furnace influence the oxygen concentration in the final crystal?
A3: Yes, modifying the heat zone design in a this compound furnace can significantly impact the oxygen concentration in the final crystal. Researchers have found that using a composite heater in a modified heat zone can effectively suppress thermal convection in the silicon melt [, ]. This suppressed convection leads to a reduced axial temperature gradient and, consequently, a lower oxygen concentration in the grown crystal [, ].
Q2: How does rapid thermal annealing (RTA) affect flow pattern defects (FPDs) in this compound?
A4: Rapid thermal annealing (RTA) has been shown to effectively reduce the density of flow pattern defects (FPDs) in this compound wafers []. Studies suggest that annealing at 1200°C for 180 seconds, particularly in a hydrogen atmosphere, leads to a significant reduction in FPD density []. This indicates that RTA can be a valuable technique for improving the quality of this compound wafers.
Q3: What is the impact of neutron irradiation on the formation of oxidation-induced stacking faults (OSFs) in this compound?
A5: Neutron irradiation can significantly hinder the formation of oxidation-induced stacking faults (OSFs) in this compound []. This suppression of OSFs is attributed to the interaction between defects introduced by neutron irradiation and oxygen atoms within the silicon lattice [].
Q4: How does heavy antimony (Sb) doping affect the oxygen concentration in this compound?
A6: Heavy antimony (Sb) doping has been shown to significantly reduce oxygen concentration in this compound [, ]. Studies using Coulometry with Electrolytic Detection by Infrared Absorption (CERDA) revealed that oxygen concentration in heavily Sb-doped this compound was about 40% lower than in lightly doped samples [, ]. This reduction is primarily attributed to the decreased solubility of oxygen in the silicon lattice when large-radius Sb atoms are introduced at high concentrations [, ].
Q5: What is the role of carbon concentration on oxygen precipitation in this compound?
A7: High carbon concentrations in this compound have a multifaceted impact on oxygen precipitation. Research shows that while high carbon content promotes oxide precipitate (OP) density [], it simultaneously inhibits the formation of thermal donors (TDs) []. Interestingly, even with increased OP density, high-carbon this compound wafers can maintain a strain-free bulk state, exhibiting minimal structural defects [].
Q6: How can this compound doped with germanium be used in electronic devices?
A8: this compound with controlled germanium doping has shown promise for developing high-performance transistors []. The presence of germanium allows for strain engineering, leading to enhanced carrier mobility in MOSFET devices []. Specifically, a compressively strained Si1-yGey layer on top of a relaxed Si1-xGex layer (where y > x) can form a high-mobility channel for holes, improving p-type MOSFET performance [].
Q7: Can this compound be used as a host material in organic light-emitting diodes (OLEDs)?
A9: While this compound itself is not typically used directly in the active layers of OLEDs, certain organic molecules incorporating silicon, particularly carbazole-based compounds containing triphenylsilyl groups, have shown promise as host materials for blue phosphorescent OLEDs [, ]. One such example is 3,6-bis(triphenylsilyl)carbazole (this compound), which, when used as a host material with the blue phosphorescent dopant FIrpic, has demonstrated high external quantum efficiencies of around 16% [].
Q8: How does the permanent magnetic field in PMCZ technology impact the growth and properties of this compound?
A10: The permanent magnetic field applied in the Permanent Magnetic Czochralski (PMCZ) method effectively suppresses thermal convection and fluctuations in the silicon melt during crystal growth [, ]. This leads to several advantages, including lower oxygen concentration, more homogenous dopant distribution, and overall improved crystal quality compared to conventionally grown this compound [, ].
Haftungsausschluss und Informationen zu In-Vitro-Forschungsprodukten
Bitte beachten Sie, dass alle Artikel und Produktinformationen, die auf BenchChem präsentiert werden, ausschließlich zu Informationszwecken bestimmt sind. Die auf BenchChem zum Kauf angebotenen Produkte sind speziell für In-vitro-Studien konzipiert, die außerhalb lebender Organismen durchgeführt werden. In-vitro-Studien, abgeleitet von dem lateinischen Begriff "in Glas", beinhalten Experimente, die in kontrollierten Laborumgebungen unter Verwendung von Zellen oder Geweben durchgeführt werden. Es ist wichtig zu beachten, dass diese Produkte nicht als Arzneimittel oder Medikamente eingestuft sind und keine Zulassung der FDA für die Vorbeugung, Behandlung oder Heilung von medizinischen Zuständen, Beschwerden oder Krankheiten erhalten haben. Wir müssen betonen, dass jede Form der körperlichen Einführung dieser Produkte in Menschen oder Tiere gesetzlich strikt untersagt ist. Es ist unerlässlich, sich an diese Richtlinien zu halten, um die Einhaltung rechtlicher und ethischer Standards in Forschung und Experiment zu gewährleisten.