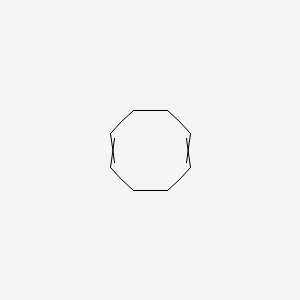
cycloocta-1,5-diene
概要
説明
cycloocta-1,5-diene, also known as (E,Z)-1,5-Cyclooctadiene, is a cyclic hydrocarbon with the chemical formula C₈H₁₂. It is one of the three configurational isomers of 1,5-Cyclooctadiene, the others being (Z,Z)-1,5-Cyclooctadiene and (E,E)-1,5-Cyclooctadiene. This compound is characterized by the presence of two double bonds in a cyclooctane ring, with one double bond in the E (trans) configuration and the other in the Z (cis) configuration .
準備方法
cycloocta-1,5-diene, can be synthesized through the dimerization of butadiene in the presence of a nickel catalyst. This process also produces vinylcyclohexene as a byproduct . The reaction conditions typically involve the use of a nickel catalyst and specific temperature and pressure settings to facilitate the dimerization process .
化学反応の分析
cycloocta-1,5-diene, undergoes various chemical reactions, including:
Oxidation: This compound can be oxidized using common oxidizing agents to form epoxides or other oxygen-containing derivatives.
Reduction: Reduction reactions can convert the double bonds into single bonds, resulting in the formation of cyclooctane.
Substitution: Nucleophilic substitution reactions can occur, where nucleophiles replace hydrogen atoms in the compound.
Cycloaddition: This compound can participate in [3+2] cycloaddition reactions with 1,3-dipoles, forming cycloadducts.
Common reagents used in these reactions include borane, sulfur dichloride, and various nucleophiles . The major products formed from these reactions depend on the specific reagents and conditions used.
科学的研究の応用
cycloocta-1,5-diene, has several scientific research applications, including:
Chemistry: It serves as a precursor to other organic compounds and is used as a ligand in organometallic chemistry.
Medicine: Its derivatives are studied for potential therapeutic applications, including drug development.
Industry: It is used in the synthesis of polymers and other industrial chemicals.
作用機序
The mechanism of action of cycloocta-1,5-diene, involves its ability to participate in various chemical reactions due to the presence of double bonds. These double bonds can undergo cycloaddition reactions, forming new chemical bonds and structures. The compound’s molecular targets and pathways depend on the specific reactions and applications being studied .
類似化合物との比較
cycloocta-1,5-diene, can be compared with its isomers, (Z,Z)-1,5-Cyclooctadiene and (E,E)-1,5-Cyclooctadiene. While all three isomers have the same chemical formula, their different configurations result in distinct chemical properties and reactivities . For example, (E,E)-1,5-Cyclooctadiene is known for its ability to perform click reactions efficiently .
Similar compounds include:
- (Z,Z)-1,5-Cyclooctadiene
- (E,E)-1,5-Cyclooctadiene
- Vinylcyclohexene (a byproduct of the dimerization process)
These compounds share similar structural features but differ in their specific configurations and chemical behaviors.
特性
分子式 |
C8H12 |
---|---|
分子量 |
108.18 g/mol |
IUPAC名 |
cycloocta-1,5-diene |
InChI |
InChI=1S/C8H12/c1-2-4-6-8-7-5-3-1/h1-2,7-8H,3-6H2 |
InChIキー |
VYXHVRARDIDEHS-UHFFFAOYSA-N |
正規SMILES |
C1CC=CCCC=C1 |
沸点 |
150.8 °C @ 757 MM HG |
Color/Form |
LIQUID |
密度 |
0.8818 @ 25 °C/4 °C |
引火点 |
95 °F 95 °F. |
melting_point |
-70 TO -69 °C |
物理的記述 |
Liquid Colorless Liquid; [MSDSonline] |
溶解性 |
INSOL IN WATER; SOL IN BENZENE, CARBON TETRACHLORIDE |
蒸気密度 |
3.66 (AIR= 1) |
蒸気圧 |
4.95 [mmHg] 0.50 PSI ABSOLUTE @ 100 °F |
製品の起源 |
United States |
Synthesis routes and methods
Procedure details
試験管内研究製品の免責事項と情報
BenchChemで提示されるすべての記事および製品情報は、情報提供を目的としています。BenchChemで購入可能な製品は、生体外研究のために特別に設計されています。生体外研究は、ラテン語の "in glass" に由来し、生物体の外で行われる実験を指します。これらの製品は医薬品または薬として分類されておらず、FDAから任何の医療状態、病気、または疾患の予防、治療、または治癒のために承認されていません。これらの製品を人間または動物に体内に導入する形態は、法律により厳格に禁止されています。これらのガイドラインに従うことは、研究と実験において法的および倫理的な基準の遵守を確実にするために重要です。