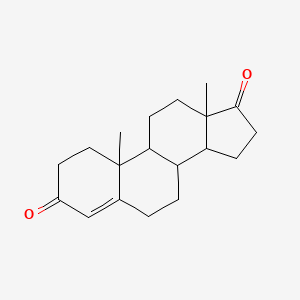
4-Androsten-3,17-dione
- 専門家チームからの見積もりを受け取るには、QUICK INQUIRYをクリックしてください。
- 品質商品を競争力のある価格で提供し、研究に集中できます。
説明
4-Androsten-3,17-dione is a useful research compound. Its molecular formula is C19H26O2 and its molecular weight is 286.4 g/mol. The purity is usually 95%.
BenchChem offers high-quality 4-Androsten-3,17-dione suitable for many research applications. Different packaging options are available to accommodate customers' requirements. Please inquire for more information about 4-Androsten-3,17-dione including the price, delivery time, and more detailed information at info@benchchem.com.
特性
分子式 |
C19H26O2 |
---|---|
分子量 |
286.4 g/mol |
IUPAC名 |
10,13-dimethyl-2,6,7,8,9,11,12,14,15,16-decahydro-1H-cyclopenta[a]phenanthrene-3,17-dione |
InChI |
InChI=1S/C19H26O2/c1-18-9-7-13(20)11-12(18)3-4-14-15-5-6-17(21)19(15,2)10-8-16(14)18/h11,14-16H,3-10H2,1-2H3 |
InChIキー |
AEMFNILZOJDQLW-UHFFFAOYSA-N |
正規SMILES |
CC12CCC(=O)C=C1CCC3C2CCC4(C3CCC4=O)C |
製品の起源 |
United States |
試験管内研究製品の免責事項と情報
BenchChemで提示されるすべての記事および製品情報は、情報提供を目的としています。BenchChemで購入可能な製品は、生体外研究のために特別に設計されています。生体外研究は、ラテン語の "in glass" に由来し、生物体の外で行われる実験を指します。これらの製品は医薬品または薬として分類されておらず、FDAから任何の医療状態、病気、または疾患の予防、治療、または治癒のために承認されていません。これらの製品を人間または動物に体内に導入する形態は、法律により厳格に禁止されています。これらのガイドラインに従うことは、研究と実験において法的および倫理的な基準の遵守を確実にするために重要です。