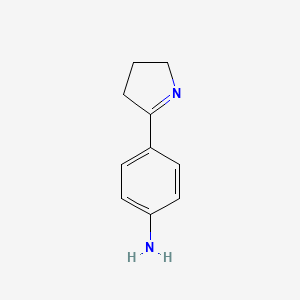
4-(3,4-dihydro-2H-pyrrol-5-yl)aniline
概要
説明
4-(3,4-dihydro-2H-pyrrol-5-yl)aniline is an organic compound that features a phenylamine group attached to a dihydropyrrole ring
準備方法
Synthetic Routes and Reaction Conditions
The synthesis of 4-(3,4-dihydro-2H-pyrrol-5-yl)aniline typically involves the reaction of a phenylamine derivative with a dihydropyrrole precursor. One common method involves the use of a palladium-catalyzed coupling reaction, where the phenylamine is reacted with a halogenated dihydropyrrole under specific conditions to form the desired compound.
Industrial Production Methods
Industrial production of this compound may involve large-scale synthesis using optimized reaction conditions to ensure high yield and purity. This often includes the use of continuous flow reactors and advanced purification techniques to isolate the compound.
化学反応の分析
Types of Reactions
4-(3,4-dihydro-2H-pyrrol-5-yl)aniline undergoes various chemical reactions, including:
Oxidation: The compound can be oxidized to form corresponding oxides.
Reduction: Reduction reactions can convert the compound into its reduced forms.
Substitution: The phenylamine group can participate in substitution reactions, where other functional groups replace hydrogen atoms.
Common Reagents and Conditions
Oxidation: Common oxidizing agents include potassium permanganate and hydrogen peroxide.
Reduction: Reducing agents such as sodium borohydride and lithium aluminum hydride are often used.
Substitution: Reagents like halogens and alkylating agents are employed under controlled conditions.
Major Products Formed
The major products formed from these reactions depend on the specific reagents and conditions used. For example, oxidation may yield oxides, while substitution reactions can produce various substituted derivatives.
科学的研究の応用
Anticancer Activity
Research indicates that compounds similar to 4-(3,4-dihydro-2H-pyrrol-5-yl)aniline exhibit significant anticancer properties. For instance, studies have shown that derivatives of pyrrole can inhibit cell proliferation in various cancer cell lines. The mechanism often involves the inhibition of key enzymes involved in cell cycle regulation, such as cyclin-dependent kinases (CDKs), which are crucial for cancer progression .
Protein Disulfide Isomerase Inhibition
This compound may also act as an inhibitor of protein disulfide isomerase (PDI), a target for developing anticancer drugs. Compounds with similar structures have demonstrated the ability to inhibit PDI activity, thus inducing apoptosis in cancer cells . The structure-activity relationship (SAR) studies further emphasize how modifications on the aniline moiety can enhance inhibitory potency .
Antimicrobial Properties
The compound's derivatives have been evaluated for their antimicrobial activities against various pathogens. Studies suggest that the incorporation of the pyrrole ring enhances the compound's ability to disrupt bacterial cell membranes or inhibit vital metabolic pathways. This opens avenues for developing new antibiotics based on this scaffold.
Organic Electronics
Due to its unique electronic properties, this compound is being explored for applications in organic electronics. The compound's ability to act as a hole transport material in organic light-emitting diodes (OLEDs) has been investigated, showing promise due to its good charge mobility and stability under operational conditions.
Photovoltaics
The compound's derivatives are also being studied as potential materials in organic photovoltaic cells. Their ability to absorb light and convert it into electrical energy makes them suitable candidates for enhancing the efficiency of solar cells.
Synthetic Routes
The synthesis of this compound can be achieved through several methods:
- Multicomponent Reactions : Combining ethyl 2,4-dioxovalerate with aromatic aldehydes and aniline under specific conditions yields high purity products .
- Cyclization Reactions : Base-assisted cyclization methods have been employed to form the pyrrole ring effectively, demonstrating good yields and selectivity .
Characterization Techniques
Characterization of synthesized compounds typically involves:
- Nuclear Magnetic Resonance (NMR) : Used for determining the structure and confirming the purity of synthesized compounds.
- Mass Spectrometry (MS) : Employed to ascertain molecular weight and structural integrity.
- X-ray Crystallography : Provides detailed information about the molecular arrangement in solid-state forms.
Case Studies
作用機序
The mechanism of action of 4-(3,4-dihydro-2H-pyrrol-5-yl)aniline involves its interaction with specific molecular targets and pathways. The compound may bind to receptors or enzymes, modulating their activity and leading to various biological effects. Detailed studies are required to elucidate the exact pathways and targets involved.
類似化合物との比較
Similar Compounds
3-(4,5-Dihydro-3H-pyrrol-2-yl)pyridine (Myosmine): A related compound with a pyridine ring instead of a phenylamine group.
Nicotine Impurity D: Another similar compound with structural similarities to 4-(3,4-dihydro-2H-pyrrol-5-yl)aniline.
Uniqueness
This compound is unique due to its specific combination of a phenylamine group and a dihydropyrrole ring, which imparts distinct chemical and biological properties compared to its analogs.
特性
分子式 |
C10H12N2 |
---|---|
分子量 |
160.22 g/mol |
IUPAC名 |
4-(3,4-dihydro-2H-pyrrol-5-yl)aniline |
InChI |
InChI=1S/C10H12N2/c11-9-5-3-8(4-6-9)10-2-1-7-12-10/h3-6H,1-2,7,11H2 |
InChIキー |
GQPLJQRKBXRVMQ-UHFFFAOYSA-N |
正規SMILES |
C1CC(=NC1)C2=CC=C(C=C2)N |
製品の起源 |
United States |
Synthesis routes and methods
Procedure details
試験管内研究製品の免責事項と情報
BenchChemで提示されるすべての記事および製品情報は、情報提供を目的としています。BenchChemで購入可能な製品は、生体外研究のために特別に設計されています。生体外研究は、ラテン語の "in glass" に由来し、生物体の外で行われる実験を指します。これらの製品は医薬品または薬として分類されておらず、FDAから任何の医療状態、病気、または疾患の予防、治療、または治癒のために承認されていません。これらの製品を人間または動物に体内に導入する形態は、法律により厳格に禁止されています。これらのガイドラインに従うことは、研究と実験において法的および倫理的な基準の遵守を確実にするために重要です。