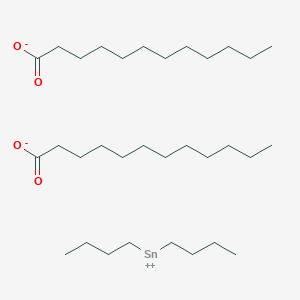
Di-n-butyldilauryltin
概要
説明
Di-n-butyldilauryltin is an organotin compound with the molecular formula C32H64O4Sn. It is a colorless, viscous, and oily liquid that is used primarily as a catalyst in various chemical reactions. The compound is known for its role in the production of polyurethanes and other polymers, where it acts as a catalyst to accelerate the reaction process.
準備方法
Synthetic Routes and Reaction Conditions: Di-n-butyldilauryltin is synthesized through the reaction of dibutyltin oxide with lauric acid. The reaction typically occurs under reflux conditions in an inert atmosphere to prevent oxidation. The general reaction is as follows:
(C4H9)2SnO+2C12H24O2→(C4H9)2Sn(O2C12H23)2+H2O
The reaction mixture is heated to remove water, and the product is purified through distillation or recrystallization.
Industrial Production Methods: In industrial settings, the production of this compound involves large-scale reactors where the reactants are mixed and heated under controlled conditions. The process is optimized to ensure high yield and purity of the final product. The use of continuous flow reactors and automated systems helps in maintaining consistent quality and efficiency.
化学反応の分析
Types of Reactions: Di-n-butyldilauryltin undergoes various types of chemical reactions, including:
Oxidation: The compound can be oxidized to form dibutyltin oxide and lauric acid.
Hydrolysis: In the presence of water, this compound hydrolyzes to form dibutyltin oxide and lauric acid.
Substitution: The laurate groups can be substituted with other carboxylates or ligands.
Common Reagents and Conditions:
Oxidation: Common oxidizing agents include hydrogen peroxide and oxygen.
Hydrolysis: Water or aqueous solutions are used under mild conditions.
Substitution: Carboxylic acids or their derivatives are used in substitution reactions.
Major Products Formed:
Oxidation: Dibutyltin oxide and lauric acid.
Hydrolysis: Dibutyltin oxide and lauric acid.
Substitution: New organotin compounds with different carboxylate groups.
科学的研究の応用
Di-n-butyldilauryltin has a wide range of applications in scientific research, including:
Chemistry: It is used as a catalyst in the synthesis of polyurethanes, polyesters, and other polymers. It also plays a role in organic synthesis reactions.
Biology: The compound is used in the study of enzyme inhibition and as a model compound for understanding the behavior of organotin compounds in biological systems.
Medicine: Research is being conducted on the potential use of this compound in drug delivery systems and as an anticancer agent.
Industry: It is widely used in the production of coatings, adhesives, and sealants due to its catalytic properties.
作用機序
The mechanism of action of di-n-butyldilauryltin involves its ability to coordinate with various substrates and catalyze reactions. The tin atom in the compound acts as a Lewis acid, facilitating the formation of intermediates and transition states in chemical reactions. This catalytic activity is crucial in accelerating the reaction rates and improving the efficiency of industrial processes.
類似化合物との比較
Dibutyltin dilaurate: Similar in structure and used as a catalyst in similar applications.
Dibutyltin oxide: Used as a precursor in the synthesis of di-n-butyldilauryltin and other organotin compounds.
Tributyltin compounds: Used in antifouling paints and as biocides, but with different applications and toxicity profiles.
Uniqueness: this compound is unique due to its specific catalytic properties and its role in the production of high-performance polymers. Its ability to act as a catalyst in a wide range of reactions makes it a valuable compound in both research and industrial applications.
特性
IUPAC Name |
dibutyltin(2+);dodecanoate | |
---|---|---|
Details | Computed by LexiChem 2.6.6 (PubChem release 2019.06.18) | |
Source | PubChem | |
URL | https://pubchem.ncbi.nlm.nih.gov | |
Description | Data deposited in or computed by PubChem | |
InChI |
InChI=1S/2C12H24O2.2C4H9.Sn/c2*1-2-3-4-5-6-7-8-9-10-11-12(13)14;2*1-3-4-2;/h2*2-11H2,1H3,(H,13,14);2*1,3-4H2,2H3;/q;;;;+2/p-2 | |
Details | Computed by InChI 1.0.5 (PubChem release 2019.06.18) | |
Source | PubChem | |
URL | https://pubchem.ncbi.nlm.nih.gov | |
Description | Data deposited in or computed by PubChem | |
InChI Key |
UKLDJPRMSDWDSL-UHFFFAOYSA-L | |
Details | Computed by InChI 1.0.5 (PubChem release 2019.06.18) | |
Source | PubChem | |
URL | https://pubchem.ncbi.nlm.nih.gov | |
Description | Data deposited in or computed by PubChem | |
Canonical SMILES |
CCCCCCCCCCCC(=O)[O-].CCCCCCCCCCCC(=O)[O-].CCCC[Sn+2]CCCC | |
Details | Computed by OEChem 2.1.5 (PubChem release 2019.06.18) | |
Source | PubChem | |
URL | https://pubchem.ncbi.nlm.nih.gov | |
Description | Data deposited in or computed by PubChem | |
Molecular Formula |
C32H64O4Sn | |
Details | Computed by PubChem 2.1 (PubChem release 2019.06.18) | |
Source | PubChem | |
URL | https://pubchem.ncbi.nlm.nih.gov | |
Description | Data deposited in or computed by PubChem | |
Molecular Weight |
631.6 g/mol | |
Details | Computed by PubChem 2.1 (PubChem release 2021.05.07) | |
Source | PubChem | |
URL | https://pubchem.ncbi.nlm.nih.gov | |
Description | Data deposited in or computed by PubChem | |
CAS No. |
77-58-7 | |
Record name | Dibutyltin dilaurate | |
Source | CAS Common Chemistry | |
URL | https://commonchemistry.cas.org/detail?cas_rn=77-58-7 | |
Description | CAS Common Chemistry is an open community resource for accessing chemical information. Nearly 500,000 chemical substances from CAS REGISTRY cover areas of community interest, including common and frequently regulated chemicals, and those relevant to high school and undergraduate chemistry classes. This chemical information, curated by our expert scientists, is provided in alignment with our mission as a division of the American Chemical Society. | |
Explanation | The data from CAS Common Chemistry is provided under a CC-BY-NC 4.0 license, unless otherwise stated. | |
Record name | DIBUTYLTIN DILAURATE | |
Source | FDA Global Substance Registration System (GSRS) | |
URL | https://gsrs.ncats.nih.gov/ginas/app/beta/substances/L4061GMT90 | |
Description | The FDA Global Substance Registration System (GSRS) enables the efficient and accurate exchange of information on what substances are in regulated products. Instead of relying on names, which vary across regulatory domains, countries, and regions, the GSRS knowledge base makes it possible for substances to be defined by standardized, scientific descriptions. | |
Explanation | Unless otherwise noted, the contents of the FDA website (www.fda.gov), both text and graphics, are not copyrighted. They are in the public domain and may be republished, reprinted and otherwise used freely by anyone without the need to obtain permission from FDA. Credit to the U.S. Food and Drug Administration as the source is appreciated but not required. | |
Retrosynthesis Analysis
AI-Powered Synthesis Planning: Our tool employs the Template_relevance Pistachio, Template_relevance Bkms_metabolic, Template_relevance Pistachio_ringbreaker, Template_relevance Reaxys, Template_relevance Reaxys_biocatalysis model, leveraging a vast database of chemical reactions to predict feasible synthetic routes.
One-Step Synthesis Focus: Specifically designed for one-step synthesis, it provides concise and direct routes for your target compounds, streamlining the synthesis process.
Accurate Predictions: Utilizing the extensive PISTACHIO, BKMS_METABOLIC, PISTACHIO_RINGBREAKER, REAXYS, REAXYS_BIOCATALYSIS database, our tool offers high-accuracy predictions, reflecting the latest in chemical research and data.
Strategy Settings
Precursor scoring | Relevance Heuristic |
---|---|
Min. plausibility | 0.01 |
Model | Template_relevance |
Template Set | Pistachio/Bkms_metabolic/Pistachio_ringbreaker/Reaxys/Reaxys_biocatalysis |
Top-N result to add to graph | 6 |
Feasible Synthetic Routes
試験管内研究製品の免責事項と情報
BenchChemで提示されるすべての記事および製品情報は、情報提供を目的としています。BenchChemで購入可能な製品は、生体外研究のために特別に設計されています。生体外研究は、ラテン語の "in glass" に由来し、生物体の外で行われる実験を指します。これらの製品は医薬品または薬として分類されておらず、FDAから任何の医療状態、病気、または疾患の予防、治療、または治癒のために承認されていません。これらの製品を人間または動物に体内に導入する形態は、法律により厳格に禁止されています。これらのガイドラインに従うことは、研究と実験において法的および倫理的な基準の遵守を確実にするために重要です。