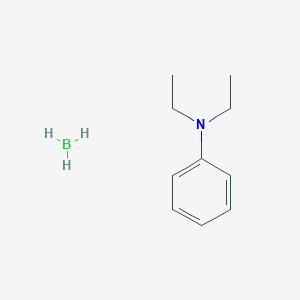
Diethylaniline-borane
概要
説明
Diethylaniline-borane (DEANB), also known as N,N-diethylaniline-borane, is a boron-amine complex with the molecular formula C₁₀H₁₈BN and a molecular weight of 160.04–160.05 g/mol . It is synthesized by combining diethylaniline (C₁₀H₁₅N) with borane (BH₃), forming a stable adduct. DEANB is a colorless to pale-yellow liquid, soluble in organic solvents like tetrahydrofuran and dichloromethane .
準備方法
Comparative Analysis of Borane Sources
DEANB vs. Borane-THF and Borane-DMS
DEANB’s advantages are highlighted in direct comparisons with conventional borane complexes :
Property | DEANB | Borane-THF | Borane-DMS |
---|---|---|---|
Stability | High | Moderate | Low |
Volatility | Low | High | Moderate |
Handling Safety | Excellent | Poor | Moderate |
Reaction Efficiency | >95% Yield | ~85% Yield | ~80% Yield |
These properties make DEANB particularly suitable for large-scale pharmaceutical applications where safety and consistency are critical.
Reaction Conditions and Optimization
Key parameters for optimizing DEANB synthesis include:
-
Temperature : Controlled exothermic reactions (25–40°C) to prevent decomposition.
-
Solvent : Ethers (e.g., THF, diethyl ether) are commonly used for their ability to stabilize borane intermediates .
-
Stoichiometry : A 1:1 molar ratio of amine to borane ensures complete adduct formation.
In the preparation of methoxy diethyl borane, reaction temperatures of 25–30°C and nitrogen atmospheres are employed to prevent oxidation . Similar conditions likely apply to DEANB.
Industrial Applications and Case Studies
Enantioselective Reduction in Pharmaceutical Synthesis
DEANB’s primary documented application is in the synthesis of (R,R)-formoterol, a long-acting β₂-agonist used in asthma treatment . The reduction of ketone 3 to bromohydrin 2 (a key intermediate) achieves >95% enantiomeric excess (ee) when using DEANB, compared to ~90% ee with borane-THF. This improvement underscores DEANB’s role in enhancing stereochemical outcomes.
化学反応の分析
Types of Reactions
Diethylaniline-borane undergoes several types of chemical reactions, primarily focusing on reduction processes. Some of the key reactions include:
Reduction: It is widely used for the reduction of ketones, acids, esters, amides, and nitriles.
Borylation: It can participate in the borylation of aryl halides.
Diastereoselective Reduction: It is used in the diastereoselective reduction of prochiral enone intermediates.
Common Reagents and Conditions
Reagents: Common reagents used with this compound include sodium borohydride, oxazaborolidine catalysts, and various solvents like tetrahydrofuran (THF).
Conditions: Reactions typically occur under mild conditions, often at room temperature or slightly elevated temperatures. The presence of catalysts can enhance the reaction efficiency and selectivity.
Major Products
The major products formed from these reactions depend on the specific substrates and conditions used. For example, the reduction of ketones typically yields secondary alcohols, while the reduction of nitriles can produce primary amines.
科学的研究の応用
Reducing Agent in Organic Synthesis
DEANB is recognized as an efficient reducing agent for a variety of functional groups. Its ability to reduce aldehydes, ketones, carboxylic acids, tertiary amides, and lactams has been extensively documented. The following table summarizes the efficacy of DEANB compared to other reducing agents:
Functional Group | DEANB Yield (%) | Other Reducing Agents Yield (%) |
---|---|---|
Aldehydes | 90-95 | 30 (Pyridine-borane) |
Ketones | 85-90 | 70 (DMS-borane) |
Carboxylic Acids | 80-85 | 60 (THF-borane) |
Tertiary Amides | 75-80 | 50 (DMS-borane) |
Lactams | 88-92 | 65 (Pyridine-borane) |
DEANB exhibits faster reaction rates and higher yields compared to traditional borane sources like THF-borane and DMS-borane, making it a preferred choice in many synthetic applications .
Hydroboration Reactions
Another significant application of DEANB is in hydroboration reactions. It has been shown to effectively add across unsaturated carbon-carbon bonds, producing organoboron compounds that can be further transformed into alcohols through oxidation.
Case Study: Hydroboration of Alkenes
In a study comparing the hydroboration efficiency of DEANB with other amine-boranes, it was found that DEANB completed the hydroboration of 1-octene significantly faster than its counterparts:
- 1-Octene Hydroboration :
- DEANB: Complete in 2 hours at room temperature.
- Pyridine-borane: 18 hours for partial reduction.
This rapid reaction time and high yield (94% for subsequent oxidation to 1-octanol) underscore DEANB's utility in organic synthesis .
Environmental Benefits
DEANB is noted for its environmental advantages over other borane reagents. It is thermally stable, easy to handle, and produces no disagreeable odors. This makes it suitable for use in settings where safety and environmental impact are critical considerations .
Catalytic Applications
Recent research has explored the use of DEANB in catalytic processes. For example, spiroborate complexes have been shown to enhance the reduction of esters and amides when combined with DEANB, increasing reaction rates and yields significantly .
Table: Catalytic Enhancements
Catalyst Type | Reaction Type | Yield Increase (%) |
---|---|---|
Spiroborate Complex | Ester Reduction | +15 |
Spiroborate Complex | Amide Reduction | +20 |
These enhancements indicate that DEANB not only serves as a reducing agent but can also improve the efficiency of various chemical transformations when used in conjunction with appropriate catalysts .
Industrial Applications
DEANB's low cost and high availability make it attractive for large-scale industrial applications, particularly in the dye industry where N,N-diethylaniline is commonly used. Its conversion to DEANB allows for efficient processing and reduction reactions essential for producing dyes and related compounds .
作用機序
The mechanism by which diethylaniline-borane exerts its effects involves the transfer of hydride ions (H-) from the borane to the substrate. This hydride transfer is facilitated by the boron atom, which acts as a Lewis acid, enhancing the nucleophilicity of the hydride. The presence of catalysts, such as oxazaborolidine, can further improve the efficiency and selectivity of the reduction process.
類似化合物との比較
Comparison with Structurally Similar Boron-Amines
Dimethylaminoborane (DMAB)
- Formula : C₂H₁₀BN | Molecular Weight : 58.92 g/mol .
- Structure : Features a smaller dimethylamine group bound to borane.
- Reactivity : Less steric hindrance compared to DEANB, enabling faster but less selective reductions. DMAB is prone to premature decomposition due to lower thermal stability .
- Applications : Primarily used in small-scale laboratory reductions.
Diethylamine Borane (DEAB)
- Formula : C₄H₁₄BN | Molecular Weight : 86.97 g/mol .
- Structure : Contains a diethylamine group instead of the aromatic diethylaniline in DEANB.
- Reactivity : Intermediate steric bulk between DMAB and DEANB. Exhibits moderate reducing power but lacks the aromatic stabilization of DEANB, leading to lower air stability .
- Applications : Industrial electrodeless coatings and niche reductions.
N-Ethyl-N-Isopropylaniline-Borane (BACH-EI™)
- Formula: Not explicitly provided | Molecular Weight: ~200 g/mol (estimated).
- Structure : Larger isopropyl and ethyl substituents on the aromatic amine.
- Reactivity : Superior hydroboration efficiency due to enhanced steric protection of the borane moiety, minimizing side reactions .
- Applications : Gold-standard reagent for hydroboration of alkenes and alkynes .
Borane-Dimethyl Sulfide (BMS)
- Formula : C₂H₉BS | Molecular Weight : 92.03 g/mol .
- Structure : Borane complexed with dimethyl sulfide instead of an amine.
- Reactivity : Less nucleophilic than DEANB due to sulfur’s weaker Lewis basicity. BMS is moisture-sensitive but widely used for BH₃ delivery in ethers .
- Applications : Precursor for borohydrides and asymmetric reductions.
Comparative Data Table
Research Findings and Industrial Relevance
- Hydrogen Storage : DEANB’s role in the five-step AB regeneration process (reduction → disproportionation → ammoniation) highlights its irreplaceability in sustainable energy systems . Competing compounds like DMAB lack the thermal stability for multi-step cycles.
- Steric Effects : DEANB’s aromatic diethylaniline group provides superior steric protection compared to aliphatic amines in DEAB or DMAB, reducing borane decomposition .
- Cost-Effectiveness : BACH-EI™ outperforms DEANB in hydroboration reactions but is prohibitively expensive for large-scale applications .
Q & A
Basic Research Questions
Q. What are the established synthetic routes for diethylaniline-borane, and how are purity and structural integrity validated?
this compound is synthesized via the disproportionation of hydridoboron catecholate, as part of a multi-step ammonia borane (AB) regeneration process. Key validation methods include nuclear magnetic resonance (NMR) spectroscopy for tracking boron-hydrogen bonding, infrared (IR) spectroscopy for functional group analysis, and X-ray crystallography for structural confirmation. Purity is assessed using gas chromatography (GC) or high-performance liquid chromatography (HPLC), with thresholds for impurities documented in peer-reviewed protocols .
Q. Which spectroscopic and computational methods are critical for characterizing this compound’s reactivity?
Dynamic NMR can monitor boron-centered stereochemical changes during reactions. Density functional theory (DFT) calculations are used to predict thermodynamic stability and reaction pathways. Coupling experimental data (e.g., kinetic studies via UV-Vis spectroscopy) with computational models ensures accurate interpretation of reactive intermediates .
Q. How should researchers design experiments to assess this compound’s role in hydrogen storage systems?
Controlled experiments should isolate variables such as temperature, pressure, and catalyst presence. For example, comparing hydrogen release rates under inert vs. catalytic conditions using mass spectrometry quantifies efficiency. Replicate trials and statistical analysis (e.g., ANOVA) minimize experimental error .
Advanced Research Questions
Q. How can discrepancies in reported thermodynamic properties of this compound be resolved?
Contradictions in enthalpy or entropy values may arise from differences in experimental conditions (e.g., solvent polarity, measurement techniques). Researchers should conduct comparative studies using standardized calorimetry methods and cross-validate results with ab initio molecular dynamics simulations. Meta-analyses of published datasets can identify systematic biases .
Q. What strategies optimize the conversion efficiency of this compound to ammonia borane (AB)?
Catalyst screening (e.g., transition metal complexes vs. organocatalysts) and reaction engineering (e.g., flow chemistry vs. batch reactors) are critical. Kinetic profiling under varying ammonia concentrations and pressure regimes can reveal rate-limiting steps. Post-reaction characterization (e.g., GC-MS) identifies byproducts affecting yield .
Q. How do solvent effects influence this compound’s stability and reactivity?
Solvent polarity and coordinating ability can alter reaction mechanisms. Systematic studies using solvents like THF, DMSO, or ionic liquids, combined with solvatochromic parameters (e.g., Kamlet-Taft), quantify solvent effects. Stability assays under inert vs. ambient conditions (monitored via NMR) guide solvent selection .
Q. What methodologies address challenges in scaling laboratory-scale this compound synthesis?
Pilot-scale studies should evaluate heat transfer, mixing efficiency, and safety protocols (e.g., exothermicity control). Process analytical technology (PAT) tools, such as in-situ Raman spectroscopy, enable real-time monitoring. Comparative life-cycle assessments (LCAs) identify bottlenecks in energy or resource use .
Q. Methodological Guidance for Data Analysis
Q. How should researchers handle inconsistent spectroscopic data in this compound studies?
Calibrate instruments using certified reference materials and validate peak assignments with isotopic labeling (e.g., deuterated analogs). Collaborative inter-laboratory studies enhance reproducibility. Data contradictions should be transparently reported, with error margins quantified using confidence intervals .
Q. What statistical approaches are recommended for analyzing reaction yield variability?
Multivariate regression models can correlate yield with experimental parameters (e.g., temperature, stoichiometry). Outlier detection methods (e.g., Grubbs’ test) identify anomalous data points. Robustness testing via Design of Experiments (DoE) frameworks optimizes reproducibility .
Q. Ethical and Reporting Standards
Q. How to ensure ethical rigor in publishing this compound research?
Disclose all conflicts of interest and raw data in supplementary materials. Follow FAIR (Findable, Accessible, Interoperable, Reusable) data principles. Cite prior work comprehensively to avoid plagiarism and contextualize findings within existing literature .
特性
IUPAC Name |
borane;N,N-diethylaniline | |
---|---|---|
Details | Computed by LexiChem 2.6.6 (PubChem release 2019.06.18) | |
Source | PubChem | |
URL | https://pubchem.ncbi.nlm.nih.gov | |
Description | Data deposited in or computed by PubChem | |
InChI |
InChI=1S/C10H15N.BH3/c1-3-11(4-2)10-8-6-5-7-9-10;/h5-9H,3-4H2,1-2H3;1H3 | |
Details | Computed by InChI 1.0.5 (PubChem release 2019.06.18) | |
Source | PubChem | |
URL | https://pubchem.ncbi.nlm.nih.gov | |
Description | Data deposited in or computed by PubChem | |
InChI Key |
YPWBYWNNJVSNPQ-UHFFFAOYSA-N | |
Details | Computed by InChI 1.0.5 (PubChem release 2019.06.18) | |
Source | PubChem | |
URL | https://pubchem.ncbi.nlm.nih.gov | |
Description | Data deposited in or computed by PubChem | |
Canonical SMILES |
B.CCN(CC)C1=CC=CC=C1 | |
Details | Computed by OEChem 2.1.5 (PubChem release 2019.06.18) | |
Source | PubChem | |
URL | https://pubchem.ncbi.nlm.nih.gov | |
Description | Data deposited in or computed by PubChem | |
Molecular Formula |
C10H18BN | |
Details | Computed by PubChem 2.1 (PubChem release 2019.06.18) | |
Source | PubChem | |
URL | https://pubchem.ncbi.nlm.nih.gov | |
Description | Data deposited in or computed by PubChem | |
Molecular Weight |
163.07 g/mol | |
Details | Computed by PubChem 2.1 (PubChem release 2021.05.07) | |
Source | PubChem | |
URL | https://pubchem.ncbi.nlm.nih.gov | |
Description | Data deposited in or computed by PubChem | |
Retrosynthesis Analysis
AI-Powered Synthesis Planning: Our tool employs the Template_relevance Pistachio, Template_relevance Bkms_metabolic, Template_relevance Pistachio_ringbreaker, Template_relevance Reaxys, Template_relevance Reaxys_biocatalysis model, leveraging a vast database of chemical reactions to predict feasible synthetic routes.
One-Step Synthesis Focus: Specifically designed for one-step synthesis, it provides concise and direct routes for your target compounds, streamlining the synthesis process.
Accurate Predictions: Utilizing the extensive PISTACHIO, BKMS_METABOLIC, PISTACHIO_RINGBREAKER, REAXYS, REAXYS_BIOCATALYSIS database, our tool offers high-accuracy predictions, reflecting the latest in chemical research and data.
Strategy Settings
Precursor scoring | Relevance Heuristic |
---|---|
Min. plausibility | 0.01 |
Model | Template_relevance |
Template Set | Pistachio/Bkms_metabolic/Pistachio_ringbreaker/Reaxys/Reaxys_biocatalysis |
Top-N result to add to graph | 6 |
Feasible Synthetic Routes
試験管内研究製品の免責事項と情報
BenchChemで提示されるすべての記事および製品情報は、情報提供を目的としています。BenchChemで購入可能な製品は、生体外研究のために特別に設計されています。生体外研究は、ラテン語の "in glass" に由来し、生物体の外で行われる実験を指します。これらの製品は医薬品または薬として分類されておらず、FDAから任何の医療状態、病気、または疾患の予防、治療、または治癒のために承認されていません。これらの製品を人間または動物に体内に導入する形態は、法律により厳格に禁止されています。これらのガイドラインに従うことは、研究と実験において法的および倫理的な基準の遵守を確実にするために重要です。