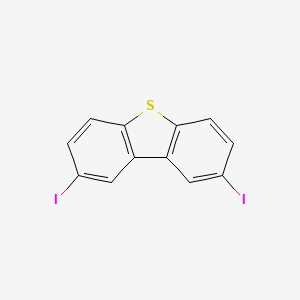
2,8-Diiododibenzothiophene
概要
説明
2,8-Diiododibenzothiophene is an organic compound with the chemical formula C12H6I2S. It is a solid substance that appears as light yellow crystals. This compound is notable for its applications in organic electronics, particularly in the development of organic light-emitting diodes (OLEDs) and organic photovoltaic cells (OPVs) due to its unique molecular structure and properties .
作用機序
Target of Action
The primary target of 2,8-Diiododibenzothiophene is the carrier mobility in wide-bandgap (WBG) Methylammonium (MA)-free based perovskite solar cells (PSCs) . The compound plays a critical role in balancing carrier transport, which is essential for efficient charge collection .
Mode of Action
This compound acts as a multifunctional additive in perovskite solar cells . It helps to overcome issues related to imbalanced carrier mobility, which can lead to non-radiative recombination loss and hinder efficient charge collection .
Biochemical Pathways
This results in enhanced photostability and improved performance of the solar cells .
Pharmacokinetics
It’s known that the compound is a solid substance at room temperature and is almost insoluble in water but soluble in organic solvents such as dichloromethane, chloroform, and ethanol .
Result of Action
The introduction of this compound in perovskite solar cells leads to enhanced photostability and a record fill factor of 85.7% . This results in improved performance of the solar cells, with a champion power conversion efficiency (PCE) of 28% achieved in 4-terminal tandem devices .
Action Environment
The action of this compound is influenced by environmental factors. For instance, the compound is sensitive to light and can undergo oxidation reactions at room temperature . It also decomposes and evaporates at high temperatures . Therefore, it’s recommended to store the compound in a dark place, sealed in dry conditions, and at a temperature of 2-8°C .
生化学分析
Biochemical Properties
2,8-Diiododibenzothiophene plays a significant role in biochemical reactions, particularly in the field of organic electronics. It interacts with various enzymes and proteins involved in electron transport and energy conversion processes. For instance, it has been shown to interact with cytochrome P450 enzymes, which are crucial for the metabolism of xenobiotics. The interaction between this compound and these enzymes involves the binding of the compound to the active site of the enzyme, leading to either inhibition or activation of the enzyme’s catalytic activity .
Cellular Effects
The effects of this compound on cellular processes are diverse. It has been observed to influence cell signaling pathways, particularly those involved in oxidative stress responses. The compound can modulate the expression of genes associated with antioxidant defense mechanisms, thereby affecting cellular metabolism. Additionally, this compound has been shown to induce apoptosis in certain cancer cell lines by disrupting mitochondrial function and triggering the release of cytochrome c .
Molecular Mechanism
At the molecular level, this compound exerts its effects through various mechanisms. One of the primary mechanisms involves the binding of the compound to specific biomolecules, such as DNA and proteins. This binding can lead to changes in the conformation and function of these biomolecules, resulting in altered gene expression and enzyme activity. For example, this compound has been shown to inhibit the activity of topoisomerase II, an enzyme involved in DNA replication and repair .
Temporal Effects in Laboratory Settings
In laboratory settings, the effects of this compound have been studied over various time periods. The compound is relatively stable under standard laboratory conditions, with minimal degradation observed over time. Prolonged exposure to light and high temperatures can lead to the breakdown of the compound, resulting in the formation of degradation products that may have different biological activities. Long-term studies have shown that this compound can have sustained effects on cellular function, particularly in terms of gene expression and metabolic activity .
Dosage Effects in Animal Models
The effects of this compound vary with different dosages in animal models. At low doses, the compound has been shown to have minimal toxic effects, while higher doses can lead to significant toxicity. For instance, high doses of this compound have been associated with liver and kidney damage in rodent models. Additionally, threshold effects have been observed, where a certain dosage level is required to elicit a biological response .
Metabolic Pathways
This compound is involved in several metabolic pathways, primarily those related to its degradation and elimination from the body. The compound is metabolized by cytochrome P450 enzymes, leading to the formation of various metabolites. These metabolites can further undergo conjugation reactions, such as glucuronidation and sulfation, to enhance their solubility and facilitate excretion. The metabolic flux of this compound and its metabolites can influence the overall levels of these compounds in the body .
Transport and Distribution
Within cells and tissues, this compound is transported and distributed through various mechanisms. The compound can interact with specific transporters and binding proteins that facilitate its movement across cell membranes. Additionally, the localization and accumulation of this compound within certain cellular compartments can affect its biological activity. For example, the compound has been shown to accumulate in the mitochondria, where it can disrupt mitochondrial function and induce apoptosis .
Subcellular Localization
The subcellular localization of this compound is crucial for its activity and function. The compound is primarily localized in the mitochondria and the endoplasmic reticulum, where it can interact with various biomolecules and influence cellular processes. Targeting signals and post-translational modifications play a role in directing this compound to specific compartments within the cell. For instance, the compound can undergo phosphorylation, which can affect its localization and activity .
準備方法
Synthetic Routes and Reaction Conditions: 2,8-Diiododibenzothiophene is typically synthesized through iodination reactions. One common method involves reacting dibenzothiophene with iodine under specific conditions to yield this compound . The reaction conditions often include the use of organic solvents such as dichloromethane or chloroform, and the reaction is carried out at elevated temperatures to facilitate the iodination process .
Industrial Production Methods: In industrial settings, the production of this compound follows similar iodination processes but on a larger scale. The use of continuous flow reactors and optimized reaction conditions ensures higher yields and purity of the final product .
化学反応の分析
Types of Reactions: 2,8-Diiododibenzothiophene undergoes various chemical reactions, including:
Substitution Reactions: The iodine atoms can be substituted with other functional groups using appropriate reagents.
Oxidation Reactions: The compound can be oxidized under specific conditions to form sulfoxides or sulfones.
Reduction Reactions: Reduction of the compound can lead to the formation of dibenzothiophene derivatives.
Common Reagents and Conditions:
Substitution: Reagents such as organometallic compounds (e.g., Grignard reagents) are commonly used.
Oxidation: Oxidizing agents like hydrogen peroxide or m-chloroperbenzoic acid are employed.
Reduction: Reducing agents such as lithium aluminum hydride or sodium borohydride are used.
Major Products:
Substitution Products: Various substituted dibenzothiophenes.
Oxidation Products: Dibenzothiophene sulfoxides and sulfones.
Reduction Products: Reduced dibenzothiophene derivatives.
科学的研究の応用
2,8-Diiododibenzothiophene has a wide range of applications in scientific research:
Chemistry: Used as a building block in the synthesis of more complex organic molecules.
Biology: Investigated for its potential interactions with biological molecules.
Medicine: Explored for its potential use in drug development and as a diagnostic tool.
Industry: Widely used in the production of organic electronic devices such as OLEDs and OPVs due to its high fluorescence intensity and efficient photoelectric conversion
類似化合物との比較
- 2,8-Dibromodibenzothiophene
- 2,8-Dichlorodibenzothiophene
- 2,8-Difluorodibenzothiophene
Comparison: 2,8-Diiododibenzothiophene is unique due to the presence of iodine atoms, which confer distinct electronic properties compared to its bromine, chlorine, and fluorine counterparts. The iodine atoms enhance the compound’s ability to participate in electron transfer processes, making it more efficient in optoelectronic applications .
特性
IUPAC Name |
2,8-diiododibenzothiophene | |
---|---|---|
Details | Computed by Lexichem TK 2.7.0 (PubChem release 2021.05.07) | |
Source | PubChem | |
URL | https://pubchem.ncbi.nlm.nih.gov | |
Description | Data deposited in or computed by PubChem | |
InChI |
InChI=1S/C12H6I2S/c13-7-1-3-11-9(5-7)10-6-8(14)2-4-12(10)15-11/h1-6H | |
Details | Computed by InChI 1.0.6 (PubChem release 2021.05.07) | |
Source | PubChem | |
URL | https://pubchem.ncbi.nlm.nih.gov | |
Description | Data deposited in or computed by PubChem | |
InChI Key |
JUMCSMONVKDUIB-UHFFFAOYSA-N | |
Details | Computed by InChI 1.0.6 (PubChem release 2021.05.07) | |
Source | PubChem | |
URL | https://pubchem.ncbi.nlm.nih.gov | |
Description | Data deposited in or computed by PubChem | |
Canonical SMILES |
C1=CC2=C(C=C1I)C3=C(S2)C=CC(=C3)I | |
Details | Computed by OEChem 2.3.0 (PubChem release 2021.05.07) | |
Source | PubChem | |
URL | https://pubchem.ncbi.nlm.nih.gov | |
Description | Data deposited in or computed by PubChem | |
Molecular Formula |
C12H6I2S | |
Details | Computed by PubChem 2.1 (PubChem release 2021.05.07) | |
Source | PubChem | |
URL | https://pubchem.ncbi.nlm.nih.gov | |
Description | Data deposited in or computed by PubChem | |
Molecular Weight |
436.05 g/mol | |
Details | Computed by PubChem 2.1 (PubChem release 2021.05.07) | |
Source | PubChem | |
URL | https://pubchem.ncbi.nlm.nih.gov | |
Description | Data deposited in or computed by PubChem | |
Retrosynthesis Analysis
AI-Powered Synthesis Planning: Our tool employs the Template_relevance Pistachio, Template_relevance Bkms_metabolic, Template_relevance Pistachio_ringbreaker, Template_relevance Reaxys, Template_relevance Reaxys_biocatalysis model, leveraging a vast database of chemical reactions to predict feasible synthetic routes.
One-Step Synthesis Focus: Specifically designed for one-step synthesis, it provides concise and direct routes for your target compounds, streamlining the synthesis process.
Accurate Predictions: Utilizing the extensive PISTACHIO, BKMS_METABOLIC, PISTACHIO_RINGBREAKER, REAXYS, REAXYS_BIOCATALYSIS database, our tool offers high-accuracy predictions, reflecting the latest in chemical research and data.
Strategy Settings
Precursor scoring | Relevance Heuristic |
---|---|
Min. plausibility | 0.01 |
Model | Template_relevance |
Template Set | Pistachio/Bkms_metabolic/Pistachio_ringbreaker/Reaxys/Reaxys_biocatalysis |
Top-N result to add to graph | 6 |
Feasible Synthetic Routes
試験管内研究製品の免責事項と情報
BenchChemで提示されるすべての記事および製品情報は、情報提供を目的としています。BenchChemで購入可能な製品は、生体外研究のために特別に設計されています。生体外研究は、ラテン語の "in glass" に由来し、生物体の外で行われる実験を指します。これらの製品は医薬品または薬として分類されておらず、FDAから任何の医療状態、病気、または疾患の予防、治療、または治癒のために承認されていません。これらの製品を人間または動物に体内に導入する形態は、法律により厳格に禁止されています。これらのガイドラインに従うことは、研究と実験において法的および倫理的な基準の遵守を確実にするために重要です。