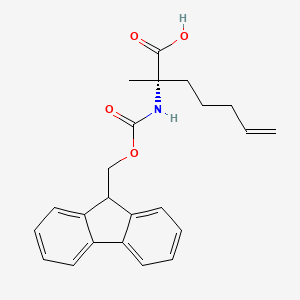
(S)-2-((((9H-Fluoren-9-yl)methoxy)carbonyl)amino)-2-methylhept-6-enoic acid
説明
(S)-2-((((9H-Fluoren-9-yl)methoxy)carbonyl)amino)-2-methylhept-6-enoic acid is a compound that features a fluorenylmethoxycarbonyl (Fmoc) protecting group attached to an amino acid derivative. This compound is commonly used in peptide synthesis due to its stability and ease of removal under mild conditions.
準備方法
Synthetic Routes and Reaction Conditions
The synthesis of (S)-2-((((9H-Fluoren-9-yl)methoxy)carbonyl)amino)-2-methylhept-6-enoic acid typically involves the protection of the amino group of the amino acid with the Fmoc group. This can be achieved using Fmoc chloride in the presence of a base such as sodium carbonate. The reaction is carried out in an organic solvent like dimethylformamide (DMF) at room temperature. The product is then purified by crystallization or chromatography .
Industrial Production Methods
Industrial production of this compound follows similar synthetic routes but on a larger scale. The process involves the use of automated peptide synthesizers that can handle multiple reactions simultaneously. The use of high-purity reagents and solvents ensures the consistency and quality of the final product.
化学反応の分析
Types of Reactions
(S)-2-((((9H-Fluoren-9-yl)methoxy)carbonyl)amino)-2-methylhept-6-enoic acid undergoes several types of chemical reactions, including:
Oxidation: The compound can be oxidized to form corresponding carbonyl compounds.
Reduction: Reduction reactions can convert the compound into its corresponding alcohols or amines.
Substitution: The Fmoc group can be substituted with other protecting groups or functional groups under appropriate conditions.
Common Reagents and Conditions
Oxidation: Common oxidizing agents include potassium permanganate (KMnO4) and chromium trioxide (CrO3).
Reduction: Reducing agents such as lithium aluminum hydride (LiAlH4) or sodium borohydride (NaBH4) are used.
Substitution: Substitution reactions often involve nucleophiles like amines or thiols in the presence of a base.
Major Products Formed
The major products formed from these reactions depend on the specific conditions and reagents used. For example, oxidation can yield ketones or aldehydes, while reduction can produce alcohols or amines.
科学的研究の応用
(S)-2-((((9H-Fluoren-9-yl)methoxy)carbonyl)amino)-2-methylhept-6-enoic acid has a wide range of applications in scientific research:
Chemistry: It is used in the synthesis of peptides and other complex molecules.
Biology: The compound is employed in the study of protein-protein interactions and enzyme mechanisms.
Medicine: It is used in the development of peptide-based drugs and therapeutic agents.
Industry: The compound is utilized in the production of specialty chemicals and materials.
作用機序
The mechanism of action of (S)-2-((((9H-Fluoren-9-yl)methoxy)carbonyl)amino)-2-methylhept-6-enoic acid involves the protection of the amino group, which prevents unwanted side reactions during peptide synthesis. The Fmoc group can be removed under mild basic conditions, typically using piperidine in DMF, to reveal the free amino group for further reactions. This selective deprotection allows for the stepwise assembly of peptides with high precision .
類似化合物との比較
Similar Compounds
(S)-2-((((9H-Fluoren-9-yl)methoxy)carbonyl)amino)-2-methylheptanoic acid: Similar structure but lacks the double bond in the hept-6-enoic acid moiety.
(S)-2-((((9H-Fluoren-9-yl)methoxy)carbonyl)amino)-2-methylhexanoic acid: Similar structure but with a shorter carbon chain.
(S)-2-((((9H-Fluoren-9-yl)methoxy)carbonyl)amino)-2-methylpentanoic acid: Similar structure but with an even shorter carbon chain.
Uniqueness
The presence of the double bond in the hept-6-enoic acid moiety of (S)-2-((((9H-Fluoren-9-yl)methoxy)carbonyl)amino)-2-methylhept-6-enoic acid provides unique reactivity and structural features that can be exploited in various chemical transformations and applications .
生物活性
(S)-2-((((9H-Fluoren-9-yl)methoxy)carbonyl)amino)-2-methylhept-6-enoic acid, commonly referred to as Fmoc-amino acid derivative, is a compound of significant interest in medicinal chemistry due to its complex structure and potential biological activities. This article provides an in-depth analysis of its biological activity, including its mechanisms of action, therapeutic applications, and relevant research findings.
Chemical Structure and Properties
The compound features a fluorenylmethoxycarbonyl (Fmoc) protective group, an amino group, and a double bond within its heptenoic acid structure. Its molecular formula is , with a molecular weight of approximately 393.45 g/mol. The stereochemistry at the 2 position contributes to its biological activity by influencing interactions with biological targets.
Biological Activity Overview
The biological activities of this compound can be categorized into several therapeutic areas:
-
Antimicrobial Activity :
- Research indicates that derivatives of fluorenone, including this compound, exhibit antimicrobial properties against various bacterial strains. The presence of the fluorenyl group is believed to enhance membrane permeability, facilitating the entry of the compound into bacterial cells and exerting its inhibitory effects on growth .
- Antitumor Activity :
- Neuroprotective Effects :
The mechanisms underlying the biological activities of this compound are complex and multifaceted:
- Inhibition of Enzymatic Activity : The compound has been shown to inhibit key enzymes involved in bacterial metabolism and replication, such as enoyl-acyl carrier protein reductase (InhA), which is crucial for fatty acid biosynthesis in bacteria .
- Cell Cycle Modulation : Research suggests that this compound can induce apoptosis in cancer cells by disrupting normal cell cycle progression and activating apoptotic pathways .
Table 1: Summary of Biological Activities
Activity Type | Mechanism | Reference |
---|---|---|
Antimicrobial | Inhibition of bacterial growth | |
Antitumor | Topoisomerase inhibition | |
Neuroprotection | Modulation of neurotransmitter systems |
Notable Studies
-
Antimicrobial Efficacy :
A study demonstrated that fluorenone derivatives exhibited significant antimicrobial activity against both Gram-positive and Gram-negative bacteria, with minimum inhibitory concentrations comparable to standard antibiotics . -
Antitumor Potential :
In vitro studies revealed that certain modifications to the fluorenone structure enhanced cytotoxicity against various cancer cell lines, suggesting a promising avenue for developing new anticancer therapeutics . -
Neuroprotective Mechanisms :
Investigations into the neuroprotective effects indicated that compounds similar to this compound can mitigate oxidative stress in neuronal cells, which is pivotal in neurodegenerative disease models .
特性
IUPAC Name |
(2S)-2-(9H-fluoren-9-ylmethoxycarbonylamino)-2-methylhept-6-enoic acid | |
---|---|---|
Source | PubChem | |
URL | https://pubchem.ncbi.nlm.nih.gov | |
Description | Data deposited in or computed by PubChem | |
InChI |
InChI=1S/C23H25NO4/c1-3-4-9-14-23(2,21(25)26)24-22(27)28-15-20-18-12-7-5-10-16(18)17-11-6-8-13-19(17)20/h3,5-8,10-13,20H,1,4,9,14-15H2,2H3,(H,24,27)(H,25,26)/t23-/m0/s1 | |
Source | PubChem | |
URL | https://pubchem.ncbi.nlm.nih.gov | |
Description | Data deposited in or computed by PubChem | |
InChI Key |
MRJFPZWLOJOINV-QHCPKHFHSA-N | |
Source | PubChem | |
URL | https://pubchem.ncbi.nlm.nih.gov | |
Description | Data deposited in or computed by PubChem | |
Canonical SMILES |
CC(CCCC=C)(C(=O)O)NC(=O)OCC1C2=CC=CC=C2C3=CC=CC=C13 | |
Source | PubChem | |
URL | https://pubchem.ncbi.nlm.nih.gov | |
Description | Data deposited in or computed by PubChem | |
Isomeric SMILES |
C[C@](CCCC=C)(C(=O)O)NC(=O)OCC1C2=CC=CC=C2C3=CC=CC=C13 | |
Source | PubChem | |
URL | https://pubchem.ncbi.nlm.nih.gov | |
Description | Data deposited in or computed by PubChem | |
Molecular Formula |
C23H25NO4 | |
Source | PubChem | |
URL | https://pubchem.ncbi.nlm.nih.gov | |
Description | Data deposited in or computed by PubChem | |
DSSTOX Substance ID |
DTXSID30670477 | |
Record name | (2S)-2-({[(9H-Fluoren-9-yl)methoxy]carbonyl}amino)-2-methylhept-6-enoic acid | |
Source | EPA DSSTox | |
URL | https://comptox.epa.gov/dashboard/DTXSID30670477 | |
Description | DSSTox provides a high quality public chemistry resource for supporting improved predictive toxicology. | |
Molecular Weight |
379.4 g/mol | |
Source | PubChem | |
URL | https://pubchem.ncbi.nlm.nih.gov | |
Description | Data deposited in or computed by PubChem | |
CAS No. |
288617-73-2 | |
Record name | (2S)-2-({[(9H-Fluoren-9-yl)methoxy]carbonyl}amino)-2-methylhept-6-enoic acid | |
Source | EPA DSSTox | |
URL | https://comptox.epa.gov/dashboard/DTXSID30670477 | |
Description | DSSTox provides a high quality public chemistry resource for supporting improved predictive toxicology. | |
試験管内研究製品の免責事項と情報
BenchChemで提示されるすべての記事および製品情報は、情報提供を目的としています。BenchChemで購入可能な製品は、生体外研究のために特別に設計されています。生体外研究は、ラテン語の "in glass" に由来し、生物体の外で行われる実験を指します。これらの製品は医薬品または薬として分類されておらず、FDAから任何の医療状態、病気、または疾患の予防、治療、または治癒のために承認されていません。これらの製品を人間または動物に体内に導入する形態は、法律により厳格に禁止されています。これらのガイドラインに従うことは、研究と実験において法的および倫理的な基準の遵守を確実にするために重要です。