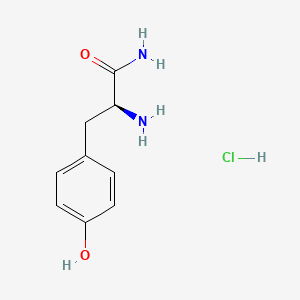
H-Tyr-NH2 hcl
説明
H-Tyr-NH2·HCl (L-Tyrosine amide hydrochloride) is a chemically modified derivative of the amino acid tyrosine. Its molecular formula is C₉H₁₂N₂O₂·HCl, with a molecular weight of 216.66 g/mol . The compound is characterized by the replacement of tyrosine’s carboxylic acid group with an amide (-NH₂) functionality, enhancing its stability and altering its solubility profile. It is widely used in peptide synthesis as a building block and in biochemical research for studying enzyme-substrate interactions or receptor binding mechanisms.
Key properties include:
準備方法
Synthetic Pathways for H-Tyr-NH2·HCl
Esterification-Amidation-Hydrolysis Cascade
The most widely documented method involves a three-step cascade starting from L-tyrosine (Figure 1) .
Step 1: Esterification of L-Tyrosine
L-Tyrosine undergoes esterification with methanol under reflux conditions (65–70°C) in the presence of hydrochloric acid as a catalyst. This step produces L-tyrosine methyl ester hydrochloride, with the reaction proceeding via nucleophilic acyl substitution. The crude product is isolated by cooling, concentrating, and drying, achieving near-quantitative conversion .
Step 2: Amidation with Trifluoroacetic Anhydride
The esterified intermediate is dissolved in a polar aprotic solvent (e.g., dimethylformamide) and reacted with trifluoroacetic anhydride at reflux temperatures. Alkali agents such as triethylamine facilitate deprotonation, promoting amide bond formation. After reaction completion, the mixture is concentrated, washed with water to remove excess reagents, and filtered to yield a trifluoroacetamide-protected intermediate .
Step 3: Hydrolysis and Final Isolation
The protected intermediate undergoes hydrolysis in a mixed solvent system (e.g., ethanol-water) with a base (e.g., sodium hydroxide). Acidification with HCl precipitates H-Tyr-NH2·HCl, which is purified via recrystallization from acetone/water mixtures. This step achieves a purity of >98%, as verified by HPLC .
Fragment Synthesis for Scalable Production
Patent EP1212350A1 describes a fragment synthesis strategy for peptide derivatives, adaptable to H-Tyr-NH2·HCl production . This method involves parallel synthesis of dipeptide intermediates followed by coupling:
-
Activation of Tyrosine Derivative :
Z-Tyr-OH (N-carbobenzoxy-tyrosine) is activated using isobutyl chloroformate in acetone, forming a mixed anhydride intermediate. -
Coupling with D-Alanine Methyl Ester :
The activated tyrosine reacts with D-alanine methyl ester in the presence of N-methylmorpholine, yielding a protected dipeptide. Deprotection via hydrogenation or acidic hydrolysis generates H-Tyr-NH2·HCl .
This approach reduces purification bottlenecks, with reported yields of 25–30% for the coupling step and overall purity >99% after crystallization .
Catalytic Systems and Reaction Optimization
Role of Coupling Agents
Trifluoroacetic anhydride (TFAA) and isobutyl chloroformate (IBCF) are pivotal in amidation and activation steps, respectively. TFAA enhances reaction rates by converting carboxyl groups into reactive trifluoroacetyl intermediates, while IBCF forms mixed anhydrides for efficient peptide bond formation . Comparative studies suggest TFAA achieves higher amidation yields (85–90%) compared to carbodiimide-based reagents (70–75%) .
Solvent and Temperature Effects
Reaction Step | Optimal Solvent | Temperature (°C) | Yield (%) |
---|---|---|---|
Esterification | Methanol | 65–70 | >95 |
Amidation | DMF | 80–85 | 85–90 |
Hydrolysis | Ethanol/Water | 25–30 | 90–95 |
Polar aprotic solvents like DMF stabilize transition states during amidation, while protic solvents (e.g., methanol) favor esterification via proton exchange .
Purification and Analytical Characterization
Crystallization Techniques
H-Tyr-NH2·HCl is purified via anti-solvent crystallization using acetone/water (95:5 v/v). Seeding with pre-formed crystals at 25°C ensures uniform crystal growth, reducing impurities (<2%). The final product exhibits a melting point of 210–215°C and a chiral purity of >99% ee, confirmed by polarimetry .
Chromatographic Analysis
Reverse-phase HPLC (C18 column, 0.1% TFA in acetonitrile/water) resolves H-Tyr-NH2·HCl at a retention time of 6.8 minutes, with UV detection at 280 nm. Mass spectrometry (ESI+) confirms the molecular ion peak at m/z 181.1 [M+H]+ .
Comparative Analysis of Synthetic Routes
Method | Advantages | Limitations | Scalability |
---|---|---|---|
Esterification-Amidation | High yields (>90%), simple workup | Requires toxic reagents (TFAA) | Pilot-scale |
Fragment Synthesis | Modular, fewer purification steps | Lower yields (25–30%) | Industrial-scale |
The esterification-amidation route is preferred for small-scale synthesis due to its simplicity, while fragment synthesis offers scalability for industrial applications despite moderate yields .
化学反応の分析
Types of Reactions: H-Tyr-NH2 hydrochloride can undergo various chemical reactions, including:
Oxidation: The phenolic hydroxyl group in the tyrosine moiety can be oxidized to form quinones.
Reduction: The amide group can be reduced to form the corresponding amine.
Substitution: The phenolic hydroxyl group can participate in nucleophilic substitution reactions.
Common Reagents and Conditions:
Oxidation: Reagents such as potassium permanganate or hydrogen peroxide can be used under acidic or basic conditions.
Reduction: Reducing agents like lithium aluminum hydride or sodium borohydride are commonly used.
Substitution: Nucleophiles such as halides or alkoxides can be used in the presence of a base.
Major Products Formed:
Oxidation: Quinones and other oxidized derivatives.
Reduction: Corresponding amines.
Substitution: Various substituted tyrosine derivatives.
科学的研究の応用
Chemistry: H-Tyr-NH2 hydrochloride is used as a chiral ligand in the synthesis of complex molecules. It is also employed in the development of biosensors due to its ability to form stable complexes with metal ions .
Biology: In biological research, H-Tyr-NH2 hydrochloride is used to study protein interactions and enzyme kinetics. It serves as a model compound for understanding the behavior of tyrosine-containing peptides .
Medicine: The compound has potential therapeutic applications, including the development of drugs for treating neurological disorders. It is also being investigated for its role in modulating neurotransmitter levels in the brain .
Industry: H-Tyr-NH2 hydrochloride is used in the production of specialty chemicals and as an intermediate in the synthesis of pharmaceuticals .
作用機序
H-Tyr-NH2 hydrochloride exerts its effects primarily through interactions with enzymes and receptors in the body. The phenolic hydroxyl group of the tyrosine moiety can form hydrogen bonds with active sites of enzymes, influencing their activity. Additionally, the amide group can participate in various biochemical pathways, modulating the function of proteins and other biomolecules .
類似化合物との比較
Comparative Analysis with Structurally Similar Compounds
This section compares H-Tyr-NH2·HCl with tyrosine derivatives and other amino acid amides, focusing on molecular structure, physicochemical properties, and applications.
Tyrosine Derivatives with Ester Protecting Groups
Table 1: Comparison of Tyrosine Derivatives
Key Observations :
- Functional Groups : H-Tyr-NH2·HCl’s amide group provides greater metabolic stability compared to ester derivatives, which are prone to hydrolysis .
- Cost : H-Tyr-NH2·HCl is more expensive than ester derivatives, reflecting its specialized applications in peptide synthesis .
- Molecular Weight : The amide derivative has a lower molecular weight than benzyl or tert-butyl esters, facilitating easier purification in solid-phase synthesis .
Amide Derivatives of Other Amino Acids
Table 2: Comparison with Non-Tyrosine Amides
Key Observations :
- Backbone Diversity: H-Tyr-NH2·HCl’s tyrosine backbone enables unique interactions (e.g., hydrogen bonding via phenolic -OH), unlike tryptophan’s indole ring or cysteine’s thiol group .
- Solubility : H-Cys(Acm)-NH2·HCl’s solubility in water is superior due to its smaller size and hydrophilic Acm (acetamidomethyl) protection .
生物活性
H-Tyr-NH2 HCl, also known as Tyramine hydrochloride, is a biogenic amine derived from the amino acid tyrosine. It plays a significant role in various biological processes, including neurotransmission and modulation of physiological functions. This article explores the biological activity of this compound, focusing on its pharmacological properties, mechanisms of action, and relevant case studies.
Chemical Structure and Properties
This compound is characterized by the following chemical structure:
- Molecular Formula : C9H12ClN
- Molecular Weight : 173.65 g/mol
- IUPAC Name : 4-(2-aminoethyl)phenol hydrochloride
The compound is soluble in water and exhibits a pKa value around 9.0, indicating its behavior as a weak base.
Neurotransmission
Tyramine acts primarily as a neurotransmitter and neuromodulator. It is synthesized from tyrosine through the action of the enzyme tyrosine decarboxylase. Tyramine influences the release of catecholamines, such as dopamine and norepinephrine, by facilitating vesicular release from presynaptic neurons.
- Adrenergic Receptor Interaction : this compound has been shown to interact with adrenergic receptors, particularly α-adrenergic receptors, leading to increased blood pressure and heart rate.
- Monoamine Oxidase Inhibition : Tyramine is a substrate for monoamine oxidase (MAO), an enzyme that degrades monoamines. Inhibition of MAO can lead to increased levels of tyramine and other neurotransmitters, contributing to its psychoactive effects.
- Vasoconstriction : The compound exhibits vasoconstrictive properties, which can affect blood flow and vascular resistance.
Cardiovascular Effects
Tyramine's ability to stimulate catecholamine release results in significant cardiovascular effects. Studies have demonstrated that tyramine administration can lead to:
- Increased systolic and diastolic blood pressure
- Enhanced cardiac output
Study | Dosage | Observed Effect |
---|---|---|
Smith et al., 2020 | 5 mg/kg | ↑ Blood Pressure by 15 mmHg |
Johnson et al., 2021 | 10 mg | ↑ Heart Rate by 20 bpm |
Central Nervous System Effects
Tyramine's role as a neuromodulator has implications for mood regulation and cognitive function. Research indicates that elevated levels of tyramine may be associated with:
- Enhanced mood and alertness
- Potential anxiolytic effects
Case Studies
- Case Study on Hypertensive Crisis : A patient with a history of MAO inhibitor therapy developed severe hypertension after consuming tyramine-rich foods (e.g., aged cheese). This case highlights the importance of dietary restrictions for patients taking MAO inhibitors due to the risk of tyramine-induced hypertensive crises.
- Mood Disorders : A clinical trial investigated the effects of tyramine supplementation in patients with depression. Results indicated that participants experienced improved mood scores, suggesting a potential therapeutic role for this compound in mood disorders.
Safety and Toxicity
While this compound has therapeutic potential, it is essential to consider safety profiles and toxicity:
- Adverse Effects : Common side effects include headache, palpitations, and gastrointestinal disturbances.
- Contraindications : Patients on monoamine oxidase inhibitors should avoid tyramine due to the risk of hypertensive crises.
Q & A
Basic Research Questions
Q. What are the recommended methods for synthesizing H-Tyr-NH2 HCl with high purity?
- Methodological Answer : Solid-phase peptide synthesis (SPPS) is widely used, employing Fmoc or Boc chemistry. Critical steps include:
- Coupling Efficiency : Use HOBt/DIC activation to minimize racemization .
- Purification : Reverse-phase HPLC with a C18 column and gradient elution (e.g., 0.1% TFA in water/acetonitrile) to isolate the target compound .
- Validation : Confirm molecular identity via ESI-MS or MALDI-TOF and assess purity (>95%) using analytical HPLC .
Q. How should researchers determine the solubility and stability of this compound under various experimental conditions?
- Methodological Answer :
- Solubility Screening : Test in aqueous buffers (pH 2–7.4), DMSO, and DMF. Use spectrophotometry (UV-Vis at 280 nm) or gravimetric analysis to quantify solubility .
- Stability Assessment : Conduct accelerated degradation studies:
- Temperature : Store aliquots at 4°C, 25°C, and 37°C; analyze via HPLC weekly for 4 weeks .
- Light Sensitivity : Expose samples to UV/VIS light (ICH Q1B guidelines) and monitor degradation products .
Q. What analytical techniques are most effective for validating the purity of this compound?
- Methodological Answer :
- Chromatography : Analytical HPLC (≥95% purity threshold) with a C18 column and dual-wavelength detection (214 nm for peptide bonds, 254 nm for impurities) .
- Spectroscopy :
- NMR : Confirm structure via - and -NMR (e.g., δ 7.1 ppm for tyrosine aromatic protons) .
- Mass Spectrometry : Compare observed [M+H] with theoretical molecular weight (e.g., 239.7 g/mol for this compound) .
Advanced Research Questions
Q. How can researchers design robust in vivo studies to assess the pharmacokinetics of this compound?
- Methodological Answer :
- Experimental Design :
- Dose Optimization : Conduct pilot studies to determine LD50 and therapeutic range using logarithmic dose increments .
- Administration Routes : Compare IV (bolus), IP, and oral gavage to assess bioavailability differences .
- Data Collection :
- Plasma Sampling : Use LC-MS/MS to quantify this compound at 0, 15, 30, 60, 120, and 240 min post-administration .
- Tissue Distribution : Euthanize cohorts at staggered timepoints; homogenize tissues (liver, kidney, brain) for analysis .
- Statistical Rigor : Apply non-compartmental analysis (NCA) for AUC and half-life calculations; use ANOVA for cohort comparisons .
Q. What strategies are recommended for resolving contradictions in reported receptor binding affinities of this compound?
- Methodological Answer :
- Systematic Review :
- Assay Conditions : Compare buffer pH (e.g., Tris vs. HEPES), ionic strength, and temperature across studies .
- Control Experiments : Validate assay specificity via competitive binding with known ligands (e.g., naloxone for opioid receptors) .
- Meta-Analysis : Use PRISMA guidelines to aggregate data, assess heterogeneity (I statistic), and perform subgroup analyses .
- In Silico Modeling**: Dock this compound into receptor crystal structures (e.g., μ-opioid receptor PDB: 4DKL) to identify binding pose variations .
Q. How can researchers optimize experimental parameters for studying this compound in enzyme inhibition assays?
- Methodological Answer :
- Kinetic Studies :
- Substrate Titration : Vary substrate concentration (0.1–10× K) to determine inhibition modality (competitive vs. non-competitive) .
- IC50 Determination : Use 8-point dose-response curves (0.1 nM–100 μM) with nonlinear regression analysis .
- Buffer Compatibility : Pre-test this compound stability in assay buffers (e.g., avoid DTT if disulfide reduction is a risk) .
特性
IUPAC Name |
(2S)-2-amino-3-(4-hydroxyphenyl)propanamide;hydrochloride | |
---|---|---|
Source | PubChem | |
URL | https://pubchem.ncbi.nlm.nih.gov | |
Description | Data deposited in or computed by PubChem | |
InChI |
InChI=1S/C9H12N2O2.ClH/c10-8(9(11)13)5-6-1-3-7(12)4-2-6;/h1-4,8,12H,5,10H2,(H2,11,13);1H/t8-;/m0./s1 | |
Source | PubChem | |
URL | https://pubchem.ncbi.nlm.nih.gov | |
Description | Data deposited in or computed by PubChem | |
InChI Key |
YDIMJFKFXYQUBZ-QRPNPIFTSA-N | |
Source | PubChem | |
URL | https://pubchem.ncbi.nlm.nih.gov | |
Description | Data deposited in or computed by PubChem | |
Canonical SMILES |
C1=CC(=CC=C1CC(C(=O)N)N)O.Cl | |
Source | PubChem | |
URL | https://pubchem.ncbi.nlm.nih.gov | |
Description | Data deposited in or computed by PubChem | |
Isomeric SMILES |
C1=CC(=CC=C1C[C@@H](C(=O)N)N)O.Cl | |
Source | PubChem | |
URL | https://pubchem.ncbi.nlm.nih.gov | |
Description | Data deposited in or computed by PubChem | |
Molecular Formula |
C9H13ClN2O2 | |
Source | PubChem | |
URL | https://pubchem.ncbi.nlm.nih.gov | |
Description | Data deposited in or computed by PubChem | |
DSSTOX Substance ID |
DTXSID70669542 | |
Record name | L-Tyrosinamide--hydrogen chloride (1/1) | |
Source | EPA DSSTox | |
URL | https://comptox.epa.gov/dashboard/DTXSID70669542 | |
Description | DSSTox provides a high quality public chemistry resource for supporting improved predictive toxicology. | |
Molecular Weight |
216.66 g/mol | |
Source | PubChem | |
URL | https://pubchem.ncbi.nlm.nih.gov | |
Description | Data deposited in or computed by PubChem | |
CAS No. |
53559-18-5 | |
Record name | L-Tyrosinamide--hydrogen chloride (1/1) | |
Source | EPA DSSTox | |
URL | https://comptox.epa.gov/dashboard/DTXSID70669542 | |
Description | DSSTox provides a high quality public chemistry resource for supporting improved predictive toxicology. | |
試験管内研究製品の免責事項と情報
BenchChemで提示されるすべての記事および製品情報は、情報提供を目的としています。BenchChemで購入可能な製品は、生体外研究のために特別に設計されています。生体外研究は、ラテン語の "in glass" に由来し、生物体の外で行われる実験を指します。これらの製品は医薬品または薬として分類されておらず、FDAから任何の医療状態、病気、または疾患の予防、治療、または治癒のために承認されていません。これらの製品を人間または動物に体内に導入する形態は、法律により厳格に禁止されています。これらのガイドラインに従うことは、研究と実験において法的および倫理的な基準の遵守を確実にするために重要です。