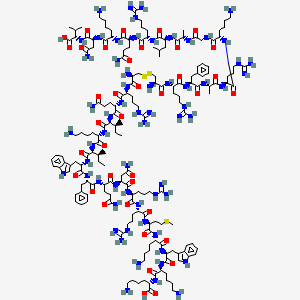
PKCβ 擬似基質
説明
PKC beta pseudosubstrate is a selective, cell-permeable inhibitor of protein kinase C beta (PKCβ). Protein kinase C is a family of phospholipid-dependent serine/threonine kinases that play a crucial role in various cellular processes, including signal transduction, cell proliferation, and differentiation. PKC beta pseudosubstrate is designed to inhibit the activity of PKCβ by mimicking its natural substrate, thereby preventing the phosphorylation of target proteins.
科学的研究の応用
PKC beta pseudosubstrate has a wide range of applications in scientific research:
Chemistry: It is used as a tool to study the structure and function of protein kinase C beta and its role in various biochemical pathways.
Biology: Researchers use PKC beta pseudosubstrate to investigate the signaling pathways mediated by PKCβ and its impact on cellular processes such as proliferation, differentiation, and apoptosis.
Medicine: The compound is employed in preclinical studies to explore its potential therapeutic effects in diseases where PKCβ is implicated, such as cancer, cardiovascular diseases, and neurological disorders.
Industry: PKC beta pseudosubstrate is utilized in the development of diagnostic assays and as a reference standard in quality control processes.
作用機序
Target of Action
The primary target of PKC beta pseudosubstrate is the Protein Kinase C (PKC) family of serine/threonine protein kinases . PKC is divided into three subfamilies based on their structural and activation characteristics: conventional or classic PKC isozymes (cPKCs; α, βI, βII, and γ), novel or non-classic PKC isozymes (nPKCs; δ, ε, η, and θ), and atypical PKC isozymes (aPKCs; ζ, ι, and λ) . PKC enzymes play a crucial role in regulating numerous cellular responses, including gene expression, protein secretion, cell proliferation, and the inflammatory response .
Mode of Action
PKC beta pseudosubstrate is a selective, cell-permeable inhibitor of PKC . It contains an auto-inhibitory pseudosubstrate domain that binds to a catalytic domain sequence to inhibit kinase activity . This interaction with its targets results in the inhibition of PKC, thereby affecting the phosphorylation of hydroxyl groups of serine and threonine amino acid residues on these proteins .
Biochemical Pathways
PKC enzymes are involved in many signaling cascades downstream of the broad class of phosphoinositide-specific phospholipases . They are responsive to the second messenger 1,2-diacylglycerol (DAG) and feature in many signaling cascades downstream of the broad class of phosphoinositide-specific phospholipases . The inhibition of PKC by PKC beta pseudosubstrate can therefore affect these biochemical pathways and their downstream effects.
Pharmacokinetics
It is known to be cell-permeable , which suggests it can be absorbed and distributed within the body’s cells.
Result of Action
The inhibition of PKC by PKC beta pseudosubstrate can lead to significant changes in the function and activity of other proteins through the phosphorylation of hydroxyl groups of serine and threonine amino acid residues on these proteins . This can result in significant physicochemical changes to the protein being phosphorylated and can be used as a means to regulate the functional activity of the modified protein .
Action Environment
The action, efficacy, and stability of PKC beta pseudosubstrate can be influenced by various environmental factors. For instance, the activation of certain PKC isoforms by DAG can be exploited in experimental systems by the use of DAG mimics . .
将来の方向性
生化学分析
Biochemical Properties
PKC Beta Pseudosubstrate is involved in the regulation of numerous cellular responses, including gene expression, protein secretion, cell proliferation, and inflammatory response . It interacts with the kinase domain, forming a hairpin structure which blocks kinase activity in the unactivated molecule . The pseudosubstrate site contains a PKC phosphorylation consensus sequence except that the phosphorylatable serine/threonine residue is replaced with an unphosphorylatable alanine .
Cellular Effects
PKC Beta Pseudosubstrate has significant effects on various types of cells and cellular processes. It influences cell function by impacting cell signaling pathways, gene expression, and cellular metabolism . For example, it is known to regulate the expression and activation of transcription factors implicated in cell cycle/mitogenesis .
Molecular Mechanism
The molecular mechanism of action of PKC Beta Pseudosubstrate involves binding interactions with biomolecules, enzyme inhibition or activation, and changes in gene expression . It contains an auto-inhibitory pseudosubstrate domain that binds a catalytic domain sequence to inhibit kinase activity .
Dosage Effects in Animal Models
The effects of PKC Beta Pseudosubstrate vary with different dosages in animal models
Metabolic Pathways
PKC Beta Pseudosubstrate is involved in several metabolic pathways. It interacts with various enzymes or cofactors
Transport and Distribution
PKC Beta Pseudosubstrate is transported and distributed within cells and tissues
準備方法
Synthetic Routes and Reaction Conditions
PKC beta pseudosubstrate is synthesized through a series of peptide synthesis steps. The compound is typically produced using solid-phase peptide synthesis (SPPS), which involves the sequential addition of protected amino acids to a growing peptide chain anchored to a solid resin. The synthesis process includes the following steps:
Resin Loading: The first amino acid is attached to the resin.
Deprotection: The protecting group on the amino acid is removed.
Coupling: The next amino acid, with its protecting group, is added and coupled to the growing peptide chain.
Repetition: Steps 2 and 3 are repeated until the desired peptide sequence is obtained.
Cleavage: The peptide is cleaved from the resin and deprotected to yield the final product.
Industrial Production Methods
Industrial production of PKC beta pseudosubstrate follows similar principles as laboratory-scale synthesis but on a larger scale. Automated peptide synthesizers are often used to increase efficiency and yield. The process involves rigorous quality control measures to ensure the purity and consistency of the final product.
化学反応の分析
Types of Reactions
PKC beta pseudosubstrate primarily undergoes peptide bond formation reactions during its synthesis. It does not typically participate in oxidation, reduction, or substitution reactions under normal conditions.
Common Reagents and Conditions
Reagents: Protected amino acids, coupling reagents (e.g., HBTU, HATU), deprotecting agents (e.g., TFA), and cleavage reagents.
Conditions: The reactions are carried out under anhydrous conditions to prevent hydrolysis. The temperature is usually maintained at room temperature, and the pH is controlled to optimize coupling efficiency.
Major Products
The major product of the synthesis is the PKC beta pseudosubstrate peptide, which is characterized by its specific amino acid sequence and molecular weight.
類似化合物との比較
PKC beta pseudosubstrate is unique in its high selectivity for PKCβ compared to other PKC inhibitors. Similar compounds include:
PKC alpha pseudosubstrate: Selective for PKCα.
PKC delta pseudosubstrate: Selective for PKCδ.
PKC epsilon pseudosubstrate: Selective for PKCε.
These compounds share a similar mechanism of action but differ in their selectivity for specific PKC isoforms, making PKC beta pseudosubstrate particularly valuable for studies focused on PKCβ.
特性
IUPAC Name |
(2S)-6-amino-2-[[(2S)-6-amino-2-[[(2S)-2-[[(2S)-6-amino-2-[[(2S)-2-[[(2S)-2-[[(2S)-2-[[(2S)-4-amino-2-[[(2S)-5-amino-2-[[(2S)-2-[[(2S)-2-[[(2S,3S)-2-[[(2S)-6-amino-2-[[(2S,3S)-2-[[(2S)-5-amino-2-[[(2S)-2-[[(2R)-2-amino-3-[[(2R)-2-amino-3-[[(2S)-1-[[(2S)-1-[[(2S)-1-[[(2S)-1-[[(2S)-6-amino-1-[[2-[[(2S)-1-[[(2S)-1-[[(2S)-1-[[(2S)-5-amino-1-[[(2S)-6-amino-1-[[(2S)-4-amino-1-[[(1S)-1-carboxy-2-methylpropyl]amino]-1,4-dioxobutan-2-yl]amino]-1-oxohexan-2-yl]amino]-1,5-dioxopentan-2-yl]amino]-5-carbamimidamido-1-oxopentan-2-yl]amino]-4-methyl-1-oxopentan-2-yl]amino]-1-oxopropan-2-yl]amino]-2-oxoethyl]amino]-1-oxohexan-2-yl]amino]-5-carbamimidamido-1-oxopentan-2-yl]amino]-1-oxopropan-2-yl]amino]-1-oxo-3-phenylpropan-2-yl]amino]-5-carbamimidamido-1-oxopentan-2-yl]amino]-3-oxopropyl]disulfanyl]propanoyl]amino]-5-carbamimidamidopentanoyl]amino]-5-oxopentanoyl]amino]-3-methylpentanoyl]amino]hexanoyl]amino]-3-methylpentanoyl]amino]-3-(1H-indol-3-yl)propanoyl]amino]-3-phenylpropanoyl]amino]-5-oxopentanoyl]amino]-4-oxobutanoyl]amino]-5-carbamimidamidopentanoyl]amino]-5-carbamimidamidopentanoyl]amino]-4-methylsulfanylbutanoyl]amino]hexanoyl]amino]-3-(1H-indol-3-yl)propanoyl]amino]hexanoyl]amino]hexanoic acid | |
---|---|---|
Source | PubChem | |
URL | https://pubchem.ncbi.nlm.nih.gov | |
Description | Data deposited in or computed by PubChem | |
InChI |
InChI=1S/C177H294N62O38S3/c1-12-96(7)140(168(272)228-114(54-28-34-73-182)159(263)238-141(97(8)13-2)169(273)236-130(86-103-90-210-109-49-23-21-47-105(103)109)165(269)232-128(84-101-44-18-15-19-45-101)163(267)226-122(63-66-134(187)241)157(261)234-131(87-136(189)243)166(270)223-119(60-40-79-207-176(199)200)149(253)220-118(59-39-78-206-175(197)198)151(255)227-124(68-81-278-11)158(262)219-112(52-26-32-71-180)153(257)233-129(85-102-89-209-108-48-22-20-46-104(102)108)164(268)221-111(51-25-31-70-179)152(256)229-125(170(274)275)55-29-35-74-183)239-160(264)123(64-67-135(188)242)225-148(252)116(57-37-76-204-173(193)194)215-144(248)106(184)92-279-280-93-107(185)145(249)216-117(58-38-77-205-174(195)196)155(259)231-127(83-100-42-16-14-17-43-100)161(265)213-99(10)143(247)214-115(56-36-75-203-172(191)192)147(251)217-110(50-24-30-69-178)146(250)211-91-138(245)212-98(9)142(246)230-126(82-94(3)4)162(266)222-120(61-41-80-208-177(201)202)150(254)224-121(62-65-133(186)240)156(260)218-113(53-27-33-72-181)154(258)235-132(88-137(190)244)167(271)237-139(95(5)6)171(276)277/h14-23,42-49,89-90,94-99,106-107,110-132,139-141,209-210H,12-13,24-41,50-88,91-93,178-185H2,1-11H3,(H2,186,240)(H2,187,241)(H2,188,242)(H2,189,243)(H2,190,244)(H,211,250)(H,212,245)(H,213,265)(H,214,247)(H,215,248)(H,216,249)(H,217,251)(H,218,260)(H,219,262)(H,220,253)(H,221,268)(H,222,266)(H,223,270)(H,224,254)(H,225,252)(H,226,267)(H,227,255)(H,228,272)(H,229,256)(H,230,246)(H,231,259)(H,232,269)(H,233,257)(H,234,261)(H,235,258)(H,236,273)(H,237,271)(H,238,263)(H,239,264)(H,274,275)(H,276,277)(H4,191,192,203)(H4,193,194,204)(H4,195,196,205)(H4,197,198,206)(H4,199,200,207)(H4,201,202,208)/t96-,97-,98-,99-,106-,107-,110-,111-,112-,113-,114-,115-,116-,117-,118-,119-,120-,121-,122-,123-,124-,125-,126-,127-,128-,129-,130-,131-,132-,139-,140-,141-/m0/s1 | |
Source | PubChem | |
URL | https://pubchem.ncbi.nlm.nih.gov | |
Description | Data deposited in or computed by PubChem | |
InChI Key |
KIWJPYSSLFMLBZ-OSCDWKIESA-N | |
Source | PubChem | |
URL | https://pubchem.ncbi.nlm.nih.gov | |
Description | Data deposited in or computed by PubChem | |
Canonical SMILES |
CCC(C)C(C(=O)NC(CCCCN)C(=O)NC(C(C)CC)C(=O)NC(CC1=CNC2=CC=CC=C21)C(=O)NC(CC3=CC=CC=C3)C(=O)NC(CCC(=O)N)C(=O)NC(CC(=O)N)C(=O)NC(CCCNC(=N)N)C(=O)NC(CCCNC(=N)N)C(=O)NC(CCSC)C(=O)NC(CCCCN)C(=O)NC(CC4=CNC5=CC=CC=C54)C(=O)NC(CCCCN)C(=O)NC(CCCCN)C(=O)O)NC(=O)C(CCC(=O)N)NC(=O)C(CCCNC(=N)N)NC(=O)C(CSSCC(C(=O)NC(CCCNC(=N)N)C(=O)NC(CC6=CC=CC=C6)C(=O)NC(C)C(=O)NC(CCCNC(=N)N)C(=O)NC(CCCCN)C(=O)NCC(=O)NC(C)C(=O)NC(CC(C)C)C(=O)NC(CCCNC(=N)N)C(=O)NC(CCC(=O)N)C(=O)NC(CCCCN)C(=O)NC(CC(=O)N)C(=O)NC(C(C)C)C(=O)O)N)N | |
Source | PubChem | |
URL | https://pubchem.ncbi.nlm.nih.gov | |
Description | Data deposited in or computed by PubChem | |
Isomeric SMILES |
CC[C@H](C)[C@@H](C(=O)N[C@@H](CCCCN)C(=O)N[C@@H]([C@@H](C)CC)C(=O)N[C@@H](CC1=CNC2=CC=CC=C21)C(=O)N[C@@H](CC3=CC=CC=C3)C(=O)N[C@@H](CCC(=O)N)C(=O)N[C@@H](CC(=O)N)C(=O)N[C@@H](CCCNC(=N)N)C(=O)N[C@@H](CCCNC(=N)N)C(=O)N[C@@H](CCSC)C(=O)N[C@@H](CCCCN)C(=O)N[C@@H](CC4=CNC5=CC=CC=C54)C(=O)N[C@@H](CCCCN)C(=O)N[C@@H](CCCCN)C(=O)O)NC(=O)[C@H](CCC(=O)N)NC(=O)[C@H](CCCNC(=N)N)NC(=O)[C@H](CSSC[C@@H](C(=O)N[C@@H](CCCNC(=N)N)C(=O)N[C@@H](CC6=CC=CC=C6)C(=O)N[C@@H](C)C(=O)N[C@@H](CCCNC(=N)N)C(=O)N[C@@H](CCCCN)C(=O)NCC(=O)N[C@@H](C)C(=O)N[C@@H](CC(C)C)C(=O)N[C@@H](CCCNC(=N)N)C(=O)N[C@@H](CCC(=O)N)C(=O)N[C@@H](CCCCN)C(=O)N[C@@H](CC(=O)N)C(=O)N[C@@H](C(C)C)C(=O)O)N)N | |
Source | PubChem | |
URL | https://pubchem.ncbi.nlm.nih.gov | |
Description | Data deposited in or computed by PubChem | |
Molecular Formula |
C177H294N62O38S3 | |
Source | PubChem | |
URL | https://pubchem.ncbi.nlm.nih.gov | |
Description | Data deposited in or computed by PubChem | |
Molecular Weight |
3995 g/mol | |
Source | PubChem | |
URL | https://pubchem.ncbi.nlm.nih.gov | |
Description | Data deposited in or computed by PubChem | |
試験管内研究製品の免責事項と情報
BenchChemで提示されるすべての記事および製品情報は、情報提供を目的としています。BenchChemで購入可能な製品は、生体外研究のために特別に設計されています。生体外研究は、ラテン語の "in glass" に由来し、生物体の外で行われる実験を指します。これらの製品は医薬品または薬として分類されておらず、FDAから任何の医療状態、病気、または疾患の予防、治療、または治癒のために承認されていません。これらの製品を人間または動物に体内に導入する形態は、法律により厳格に禁止されています。これらのガイドラインに従うことは、研究と実験において法的および倫理的な基準の遵守を確実にするために重要です。