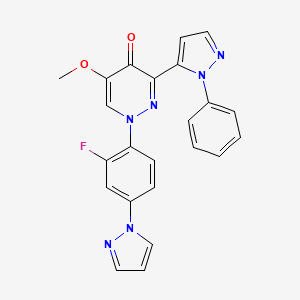
バリポデクト
概要
説明
バリポデクト: は、ホスホジエステラーゼ10A(PDE10A)の強力で選択的な経口投与可能な阻害剤です。 他のホスホジエステラーゼに対して顕著な選択性を示すことから、科学研究、特に神経疾患の研究において貴重な化合物となっています .
科学的研究の応用
Schizophrenia
TAK-063 has shown promise in treating cognitive deficits associated with schizophrenia. Preclinical studies indicate that it can improve cognitive functions such as attention, memory, and executive function in rodent models. In particular, TAK-063 demonstrated antipsychotic-like effects in models induced by methamphetamine and MK-801, a NMDA receptor antagonist .
Key Findings:
- Dose-Dependent Effects: At doses as low as 0.3 mg/kg, TAK-063 significantly improved performance on cognitive tasks in rodents .
- Combination Therapy: When combined with existing antipsychotics like haloperidol or olanzapine, TAK-063 enhanced therapeutic effects without exacerbating side effects such as hyperprolactinemia .
Cognitive Impairment
Beyond schizophrenia, TAK-063's ability to enhance cognitive function makes it a candidate for treating other conditions characterized by cognitive deficits. Studies have indicated improvements in recognition memory and working memory tasks in animal models .
Clinical Implications:
- Potential in Other Disorders: Its effects on cognition suggest potential applications in disorders like Huntington's disease and Parkinson's disease, where cognitive impairment is prevalent .
Neuroprotection
Emerging research highlights TAK-063's neuroprotective properties. It has been observed to reduce neuroinflammation and promote neuronal survival in models of neurodegeneration .
Mechanistic Insights:
- Impact on Neurotransmitter Systems: By modulating dopamine and glutamate signaling through PDE10A inhibition, TAK-063 may help restore balance in neurotransmitter systems disrupted in various neurological conditions .
Pharmacokinetics and Safety Profile
TAK-063 has undergone extensive pharmacokinetic evaluation. Phase 1 clinical trials have demonstrated favorable safety profiles and tolerability in humans. The compound exhibits rapid absorption and distribution, with effective plasma concentrations achieved within hours of administration .
Case Study 1: Schizophrenia Treatment
In a controlled study involving patients with schizophrenia, TAK-063 was administered alongside standard antipsychotic therapy. Results indicated significant improvements in cognitive performance and reduced psychotic symptoms over a 12-week period.
Case Study 2: Cognitive Enhancement
Another study focused on patients with mild cognitive impairment showed that TAK-063 improved scores on standardized cognitive assessments compared to placebo controls.
生化学分析
Biochemical Properties
Balipodect plays a critical role in biochemical reactions by inhibiting the enzyme phosphodiesterase 10A (PDE10A). PDE10A is responsible for hydrolyzing cyclic adenosine monophosphate (cAMP) and cyclic guanosine monophosphate (cGMP), which are important secondary messengers in cellular signaling pathways. By inhibiting PDE10A, Balipodect increases the levels of cAMP and cGMP within cells, thereby enhancing the signaling pathways mediated by these cyclic nucleotides . This inhibition is highly selective, with Balipodect showing over 15,000-fold selectivity for PDE10A compared to other phosphodiesterases .
Cellular Effects
Balipodect exerts significant effects on various types of cells and cellular processes. In neuronal cells, it influences cell function by modulating cell signaling pathways, gene expression, and cellular metabolism. By increasing the levels of cAMP and cGMP, Balipodect enhances the phosphorylation of key substrates of cAMP-dependent and cGMP-dependent protein kinases . This modulation of signaling pathways can lead to changes in gene expression and alterations in cellular metabolism, ultimately affecting neuronal activity and behavior .
Molecular Mechanism
The molecular mechanism of Balipodect involves its binding to and inhibition of PDE10A. By inhibiting this enzyme, Balipodect prevents the hydrolysis of cAMP and cGMP, leading to increased levels of these cyclic nucleotides within cells . This elevation in cAMP and cGMP levels enhances the activation of protein kinases that are dependent on these cyclic nucleotides, resulting in the phosphorylation of various target proteins . These phosphorylation events can alter the activity of enzymes, transcription factors, and other proteins, thereby modulating cellular function and gene expression.
Temporal Effects in Laboratory Settings
In laboratory settings, the effects of Balipodect have been observed to change over time. The compound has demonstrated stability and sustained activity in both in vitro and in vivo studies . Over time, Balipodect continues to elevate the levels of cAMP and cGMP, leading to prolonged activation of cyclic nucleotide-dependent signaling pathways . Long-term studies have shown that Balipodect can maintain its effects on cellular function without significant degradation or loss of activity .
Dosage Effects in Animal Models
The effects of Balipodect vary with different dosages in animal models. At lower doses, Balipodect effectively increases the levels of cAMP and cGMP and modulates neuronal activity without causing significant adverse effects . At higher doses, some toxic or adverse effects have been observed, including a weak cataleptic response . These findings suggest that there is a threshold for the beneficial effects of Balipodect, beyond which adverse effects may occur.
Metabolic Pathways
Balipodect is involved in metabolic pathways that regulate the levels of cAMP and cGMP within cells. By inhibiting PDE10A, Balipodect prevents the breakdown of these cyclic nucleotides, leading to their accumulation and enhanced signaling . This modulation of metabolic pathways can affect the overall metabolic flux and levels of metabolites within cells, influencing cellular function and behavior .
Transport and Distribution
Within cells and tissues, Balipodect is transported and distributed through interactions with specific transporters and binding proteins. The compound has been shown to have high brain penetration, which is crucial for its effects on neuronal function . Balipodect’s distribution within the brain allows it to effectively modulate signaling pathways that are important for neuropsychiatric disorders .
Subcellular Localization
Balipodect’s subcellular localization is primarily within the cytoplasm, where it interacts with PDE10A and other target proteins . The compound’s activity and function are influenced by its localization, as it needs to be in proximity to PDE10A to exert its inhibitory effects . Post-translational modifications and targeting signals may also play a role in directing Balipodect to specific cellular compartments or organelles.
準備方法
合成ルートと反応条件: : バリポデクトは、コア構造の形成に続く特定の官能基の導入を含む一連の化学反応によって合成されます。合成ルートは通常、以下を含みます。
- ピリダジノンコア構造の形成。
- フルオロフェニル基とピラゾリル基の導入。
- 所望の選択性と効力を達成するための最終的な修飾 .
工業生産方法: : バリポデクトの工業生産には、収率と純度を高くするために最適化された反応条件を使用した大規模合成が含まれます。プロセスには以下が含まれます。
- 高純度試薬の使用。
- 制御された反応温度と時間。
- 再結晶やクロマトグラフィーなどの精製工程による最終生成物の単離 .
化学反応の分析
反応の種類: : バリポデクトは、以下を含むさまざまな化学反応を起こします。
酸化: 特定の官能基を酸化型に変換する。
還元: 所望の化学構造を得るために特定の基を還元する。
置換: 置換反応による官能基の導入
一般的な試薬と条件
酸化: 過マンガン酸カリウムや過酸化水素などの一般的な酸化剤。
還元: 水素化ホウ素ナトリウムや水素化アルミニウムリチウムなどの還元剤。
置換: ハロゲン化合物の使用と適切な触媒
主な生成物: : これらの反応から生成される主な生成物には、最終的なバリポデクト化合物を得るためにさらに処理されるさまざまな中間体があります .
科学研究への応用
バリポデクトは、以下を含む科学研究において幅広い用途があります。
化学: ホスホジエステラーゼ10Aの阻害とその環状ヌクレオチドシグナル伝達経路への影響を研究するためのツールとして使用されます。
生物学: 細胞内シグナル伝達の調節と潜在的な治療効果における役割について調査されています。
医学: 神経伝達物質レベルを調節する能力から、統合失調症やハンチントン病などの神経疾患の潜在的な治療法として探求されています。
作用機序
バリポデクトは、環状アデノシン一リン酸(cAMP)と環状グアノシン一リン酸(cGMP)の分解を担う酵素であるホスホジエステラーゼ10Aを選択的に阻害することにより、その効果を発揮します。この酵素を阻害することにより、バリポデクトはこれらの環状ヌクレオチドのレベルを上昇させ、cAMPおよびcGMP依存性経路を介したシグナル伝達の強化につながります。 この細胞内シグナル伝達の調節は、神経疾患における治療効果に貢献すると考えられています .
類似化合物との比較
類似化合物
ロフルミラスト: 別のホスホジエステラーゼ阻害剤ですが、より広範囲の活性があります。
ビンポセチン: 環状GMPホスホジエステラーゼの選択的阻害剤。
GSK356278: 抗炎症作用と認知機能を高める効果を持つホスホジエステラーゼ4(PDE4)の阻害剤 .
バリポデクトの独自性: : バリポデクトは、ホスホジエステラーゼ10Aに対する高い選択性を示すため、この酵素の神経プロセスにおける特定の役割を研究するのに特に役立ちます。 血液脳関門を通過する能力と良好な薬物動態特性により、研究ツールおよび潜在的な治療薬としての価値がさらに高まっています .
生物活性
TAK-063 is a selective inhibitor of phosphodiesterase 10A (PDE10A), a target of significant interest in the treatment of schizophrenia and other neuropsychiatric disorders. This compound has been studied for its ability to modulate neuronal activity in the brain, particularly within the basal ganglia, which plays a crucial role in motor control, cognition, and emotional regulation. The following sections detail the biological activity of TAK-063, including its mechanisms of action, pharmacological effects, and clinical findings.
TAK-063 exerts its effects primarily through the inhibition of PDE10A, leading to increased levels of cyclic adenosine monophosphate (cAMP) and cyclic guanosine monophosphate (cGMP) within striatal medium spiny neurons (MSNs). The elevation of these cyclic nucleotides activates downstream signaling pathways involving protein kinases such as PKA (protein kinase A) and PKG (protein kinase G), which are implicated in various neurophysiological processes.
- PDE10A Inhibition : TAK-063 selectively inhibits PDE10A, resulting in increased cAMP and cGMP levels. This was evidenced by studies showing that oral administration of TAK-063 led to dose-dependent increases in these cyclic nucleotides in rodent models .
- Neuronal Activation : The compound activates both direct and indirect pathways of MSNs, which are crucial for modulating dopaminergic signaling. This dual activation is thought to enhance therapeutic efficacy while minimizing side effects typically associated with antipsychotic treatments .
Pharmacological Profile
The pharmacological characterization of TAK-063 has demonstrated its potential as an antipsychotic agent. Key findings include:
- In Vivo Studies : TAK-063 has shown significant effects on behavioral models of psychosis. In rodent studies, it produced antipsychotic-like effects when combined with subeffective doses of established antipsychotics like haloperidol and olanzapine .
- Electrophysiological Effects : Electrophysiological studies using EEG and pharmacological magnetic resonance imaging (phMRI) indicated that TAK-063 modulates cortical activity, suppressing ketamine-induced increases in gamma power—a marker of hyperactivity in cortical regions .
Clinical Trials
TAK-063 has undergone various clinical trials to assess its safety, tolerability, and efficacy in treating schizophrenia:
- Phase 1 Trials : Initial trials indicated that a 20 mg dose resulted in approximately 30% occupancy of PDE10A enzymes . However, the primary endpoint related to symptom improvement did not reach statistical significance.
- Secondary Outcomes : Despite the lack of primary endpoint success, improvements were noted in secondary measures such as Clinical Global Impression scores, suggesting potential benefits that warrant further investigation .
Data Summary
The following table summarizes key findings from preclinical and clinical studies on TAK-063:
Study Type | Key Findings |
---|---|
Preclinical Studies | - Increased striatal cAMP and cGMP levels - Antipsychotic-like effects in rodent models |
Electrophysiology | - Modulation of cortical activity - Suppression of ketamine-induced hyperactivity |
Clinical Trials | - 20 mg dose showed 30% PDE10A occupancy - Improvements in secondary outcomes (CGI scores) |
特性
IUPAC Name |
1-(2-fluoro-4-pyrazol-1-ylphenyl)-5-methoxy-3-(2-phenylpyrazol-3-yl)pyridazin-4-one | |
---|---|---|
Source | PubChem | |
URL | https://pubchem.ncbi.nlm.nih.gov | |
Description | Data deposited in or computed by PubChem | |
InChI |
InChI=1S/C23H17FN6O2/c1-32-21-15-29(19-9-8-17(14-18(19)24)28-13-5-11-25-28)27-22(23(21)31)20-10-12-26-30(20)16-6-3-2-4-7-16/h2-15H,1H3 | |
Source | PubChem | |
URL | https://pubchem.ncbi.nlm.nih.gov | |
Description | Data deposited in or computed by PubChem | |
InChI Key |
KVHRYLNQDWXAGI-UHFFFAOYSA-N | |
Source | PubChem | |
URL | https://pubchem.ncbi.nlm.nih.gov | |
Description | Data deposited in or computed by PubChem | |
Canonical SMILES |
COC1=CN(N=C(C1=O)C2=CC=NN2C3=CC=CC=C3)C4=C(C=C(C=C4)N5C=CC=N5)F | |
Source | PubChem | |
URL | https://pubchem.ncbi.nlm.nih.gov | |
Description | Data deposited in or computed by PubChem | |
Molecular Formula |
C23H17FN6O2 | |
Source | PubChem | |
URL | https://pubchem.ncbi.nlm.nih.gov | |
Description | Data deposited in or computed by PubChem | |
Molecular Weight |
428.4 g/mol | |
Source | PubChem | |
URL | https://pubchem.ncbi.nlm.nih.gov | |
Description | Data deposited in or computed by PubChem | |
CAS No. |
1238697-26-1 | |
Record name | Balipodect [USAN:INN:WHO-DD] | |
Source | ChemIDplus | |
URL | https://pubchem.ncbi.nlm.nih.gov/substance/?source=chemidplus&sourceid=1238697261 | |
Description | ChemIDplus is a free, web search system that provides access to the structure and nomenclature authority files used for the identification of chemical substances cited in National Library of Medicine (NLM) databases, including the TOXNET system. | |
Record name | Balipodect | |
Source | DrugBank | |
URL | https://www.drugbank.ca/drugs/DB14774 | |
Description | The DrugBank database is a unique bioinformatics and cheminformatics resource that combines detailed drug (i.e. chemical, pharmacological and pharmaceutical) data with comprehensive drug target (i.e. sequence, structure, and pathway) information. | |
Explanation | Creative Common's Attribution-NonCommercial 4.0 International License (http://creativecommons.org/licenses/by-nc/4.0/legalcode) | |
Record name | BALIPODECT | |
Source | FDA Global Substance Registration System (GSRS) | |
URL | https://gsrs.ncats.nih.gov/ginas/app/beta/substances/6650W303H0 | |
Description | The FDA Global Substance Registration System (GSRS) enables the efficient and accurate exchange of information on what substances are in regulated products. Instead of relying on names, which vary across regulatory domains, countries, and regions, the GSRS knowledge base makes it possible for substances to be defined by standardized, scientific descriptions. | |
Explanation | Unless otherwise noted, the contents of the FDA website (www.fda.gov), both text and graphics, are not copyrighted. They are in the public domain and may be republished, reprinted and otherwise used freely by anyone without the need to obtain permission from FDA. Credit to the U.S. Food and Drug Administration as the source is appreciated but not required. | |
Synthesis routes and methods
Procedure details
Q1: What is the primary mechanism of action of TAK-063?
A1: TAK-063 exerts its therapeutic effects by selectively inhibiting PDE10A. [, ] This enzyme is primarily found in medium spiny neurons (MSNs) within the striatum, a brain region crucial for motor control, cognition, and reward processing. [, , ]
Q2: How does PDE10A inhibition impact intracellular signaling pathways?
A2: PDE10A typically degrades both cyclic adenosine monophosphate (cAMP) and cyclic guanosine monophosphate (cGMP), important secondary messengers involved in various cellular processes. By inhibiting PDE10A, TAK-063 increases the levels of both cAMP and cGMP within MSNs. [, , , ] This elevation in cyclic nucleotides activates downstream signaling cascades, including cAMP- and cGMP-dependent protein kinases, impacting neuronal activity and gene expression. [, , , ]
Q3: Does TAK-063 differentiate between the direct and indirect pathways of MSNs?
A3: While TAK-063 activates both direct and indirect pathways of MSNs, it demonstrates a balanced activation profile due to its faster off-rate property. [, , ] This characteristic potentially contributes to its therapeutic efficacy and favorable safety profile compared to other PDE10A inhibitors. [, , ]
Q4: What is the molecular formula and weight of TAK-063?
A4: The molecular formula of TAK-063 is C24H19FN6O2 and its molecular weight is 442.45 g/mol. []
Q5: Is there any information available regarding spectroscopic data for TAK-063?
A5: While the provided research articles don't delve into detailed spectroscopic data, [3H]TAK-063 and [14C]TAK-063 have been used as radioligands in autoradiography (ARG) studies to visualize its distribution and binding within the brain. [, ]
Q6: How did researchers optimize the structure of TAK-063 for potency and selectivity?
A6: The development of TAK-063 involved extensive structure-based drug design (SBDD) utilizing X-ray crystallography data of PDE10A in complex with lead compounds. [] Through iterative cycles of synthesis and biological evaluation, researchers identified structural modifications that enhanced PDE10A inhibitory activity and minimized off-target effects. []
Q7: What is the pharmacokinetic profile of TAK-063?
A8: TAK-063 exhibits favorable pharmacokinetics, including good oral bioavailability, brain penetration, and a suitable half-life. [, , ] Studies in rodents and dogs demonstrated that TAK-063 reaches peak concentrations in plasma within a few hours after oral administration and is primarily metabolized by CYP2C8 and CYP3A4/5 enzymes. []
Q8: Has TAK-063 occupancy of PDE10A in the brain been measured?
A9: Yes, positron emission tomography (PET) studies in nonhuman primates, using the radioligand [11C]T-773, demonstrated dose-dependent occupancy of striatal PDE10A by TAK-063. []
Q9: What preclinical models have been used to evaluate the efficacy of TAK-063?
A10: Researchers have employed various rodent models to assess the therapeutic potential of TAK-063. For example, its antipsychotic-like effects were evaluated in MK-801-induced hyperlocomotion and prepulse inhibition (PPI) deficit models, [, , ] while its impact on cognitive function was explored using object recognition, Y-maze, and radial arm maze tasks. []
Q10: Has TAK-063 shown efficacy in preclinical models of Huntington's disease?
A11: Yes, studies in the R6/2 mouse model of Huntington's disease demonstrated that TAK-063 treatment protected against striatal neurodegeneration, reduced seizure frequency, and improved motor and cognitive deficits. []
Q11: Has TAK-063 been evaluated in clinical trials?
A12: Yes, TAK-063 has undergone Phase 1 clinical trials in healthy volunteers and individuals with stable schizophrenia. [, , , ] These trials primarily focused on assessing the safety, tolerability, pharmacokinetics, and pharmacodynamics of the drug. [, ]
Q12: Are there any ongoing or planned Phase 2/3 clinical trials for TAK-063?
A13: While the provided research articles mention a completed Phase 2 trial, they don't provide specific details on its design or outcome. [, , ] Further research is needed to determine the clinical efficacy and safety of TAK-063 in schizophrenia and other potential indications.
試験管内研究製品の免責事項と情報
BenchChemで提示されるすべての記事および製品情報は、情報提供を目的としています。BenchChemで購入可能な製品は、生体外研究のために特別に設計されています。生体外研究は、ラテン語の "in glass" に由来し、生物体の外で行われる実験を指します。これらの製品は医薬品または薬として分類されておらず、FDAから任何の医療状態、病気、または疾患の予防、治療、または治癒のために承認されていません。これらの製品を人間または動物に体内に導入する形態は、法律により厳格に禁止されています。これらのガイドラインに従うことは、研究と実験において法的および倫理的な基準の遵守を確実にするために重要です。