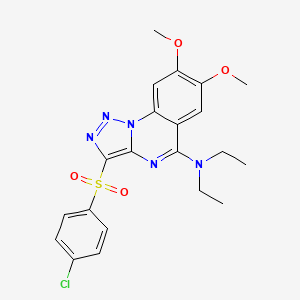
タルゴシル
概要
説明
Targocil is a bacteriostatic inhibitor that targets the biosynthesis of wall teichoic acid, a crucial component of the cell wall in Gram-positive bacteria. It is particularly effective against methicillin-susceptible Staphylococcus aureus and methicillin-resistant Staphylococcus aureus, with a minimum inhibitory concentration of 2 micrograms per milliliter for both strains .
科学的研究の応用
Targocil has a wide range of applications in scientific research:
Chemistry: Used as a tool to study the biosynthesis of wall teichoic acid and its role in bacterial cell wall integrity.
Biology: Employed in research on bacterial resistance mechanisms, particularly in methicillin-resistant Staphylococcus aureus.
Medicine: Investigated for its potential as an antibacterial agent against resistant bacterial strains.
Industry: Utilized in the development of new antibacterial coatings and materials.
作用機序
Target of Action
Targocil is a novel agent that targets the TarG subunit of the Wall Teichoic Acid (WTA) translocase (TarGH) . This translocase is responsible for transporting WTA across the membrane to the wall .
Mode of Action
Targocil functions as a bacteriostatic inhibitor of WTA biosynthesis . It interacts with TarG and inhibits the TarGH complex that translocates WTA across the membrane . This interaction results in a significant decrease in autolysis .
Biochemical Pathways
The primary biochemical pathway affected by Targocil is the WTA biosynthesis pathway . WTA is a major component of the cell wall of Gram-positive bacteria like Staphylococcus aureus . By inhibiting the TarGH complex, Targocil effectively blocks the translocation of WTA across the membrane, disrupting the WTA biosynthesis pathway .
Result of Action
The inhibition of the TarGH complex by Targocil leads to a rapid shutdown of cellular autolysis in methicillin-susceptible Staphylococcus aureus strains . This is due to the decreased translocation of the major autolysin Atl across the membrane .
Action Environment
The efficacy of Targocil can be influenced by the presence of resistance mechanisms in bacteria. For instance, resistance to Targocil can be achieved by loss-of-function mutations in tarO or tarA, which effectively bypass the mechanism of action of Targocil . Therefore, the genetic makeup of the bacterial strain can significantly influence the action, efficacy, and stability of Targocil.
Safety and Hazards
生化学分析
Biochemical Properties
Targocil interacts with the ABC transporter TarGH, inhibiting its function . This interaction disrupts the transport of WTA, affecting the biochemical reactions associated with cell wall synthesis in Gram-positive bacteria .
Cellular Effects
Targocil’s inhibition of TarGH affects the function of cells, particularly methicillin-resistant Staphylococcus aureus (MRSA), by disrupting the synthesis of the cell wall . This can impact cell signaling pathways, gene expression, and cellular metabolism .
Molecular Mechanism
Targocil exerts its effects at the molecular level by binding to the ABC transporter TarGH, inhibiting its function . This results in the disruption of WTA transport, affecting cell wall synthesis and leading to changes in gene expression .
Temporal Effects in Laboratory Settings
The effects of Targocil on TarGH and, consequently, on cell wall synthesis, can be observed over time in laboratory settings
Metabolic Pathways
Targocil’s primary interaction is with the ABC transporter TarGH, which is involved in the transport of WTA, a key component in the metabolic pathways of cell wall synthesis .
Transport and Distribution
Targocil targets the ABC transporter TarGH, affecting the transport and distribution of WTA within cells . This can impact the localization or accumulation of WTA in the cell wall .
Subcellular Localization
Targocil’s primary target, the ABC transporter TarGH, is located in the cell membrane . The inhibition of TarGH by Targocil affects the transport of WTA, impacting its subcellular localization .
準備方法
Synthetic Routes and Reaction Conditions: The synthesis of Targocil involves multiple steps, starting with the preparation of the quinoline moiety, which is then functionalized with various substituents. The key steps include:
Formation of the Quinoline Core: This is typically achieved through a Skraup synthesis, involving the condensation of aniline with glycerol in the presence of sulfuric acid and an oxidizing agent.
Functionalization: The quinoline core is then functionalized with methoxy and chloro substituents through electrophilic aromatic substitution reactions.
Final Assembly: The functionalized quinoline is coupled with a benzene derivative to form the final Targocil structure.
Industrial Production Methods: Industrial production of Targocil follows similar synthetic routes but on a larger scale, with optimizations for yield and purity. The process involves:
Batch Reactions: Conducted in large reactors with precise control over temperature, pressure, and reaction time.
Purification: The crude product is purified using techniques such as recrystallization and chromatography to achieve high purity levels.
化学反応の分析
Types of Reactions: Targocil undergoes various chemical reactions, including:
Oxidation: Targocil can be oxidized under specific conditions, leading to the formation of quinoline N-oxide derivatives.
Reduction: Reduction of Targocil can yield dihydroquinoline derivatives.
Substitution: Electrophilic and nucleophilic substitution reactions can modify the functional groups on the quinoline and benzene rings.
Common Reagents and Conditions:
Oxidation: Hydrogen peroxide or peracids in the presence of a catalyst.
Reduction: Hydrogen gas with a palladium catalyst or sodium borohydride.
Substitution: Halogenating agents for electrophilic substitution and nucleophiles like amines for nucleophilic substitution.
Major Products:
Oxidation Products: Quinoline N-oxides.
Reduction Products: Dihydroquinolines.
Substitution Products: Various substituted quinoline and benzene derivatives.
類似化合物との比較
1835F03: A precursor to Targocil with similar antibacterial activity but lower potency.
Clomiphene: An inhibitor of undecaprenyl diphosphate synthase, another enzyme involved in bacterial cell wall biosynthesis.
Uniqueness of Targocil: Targocil is unique in its specific targeting of the TarG subunit of the wall teichoic acid translocase, making it highly effective against methicillin-resistant Staphylococcus aureus. Its ability to inhibit both methicillin-susceptible and methicillin-resistant strains at low concentrations sets it apart from other antibacterial agents.
特性
IUPAC Name |
3-(4-chlorophenyl)sulfonyl-N,N-diethyl-7,8-dimethoxytriazolo[1,5-a]quinazolin-5-amine | |
---|---|---|
Source | PubChem | |
URL | https://pubchem.ncbi.nlm.nih.gov | |
Description | Data deposited in or computed by PubChem | |
InChI |
InChI=1S/C21H22ClN5O4S/c1-5-26(6-2)19-15-11-17(30-3)18(31-4)12-16(15)27-20(23-19)21(24-25-27)32(28,29)14-9-7-13(22)8-10-14/h7-12H,5-6H2,1-4H3 | |
Source | PubChem | |
URL | https://pubchem.ncbi.nlm.nih.gov | |
Description | Data deposited in or computed by PubChem | |
InChI Key |
TYNZGYMGTTUYKZ-UHFFFAOYSA-N | |
Source | PubChem | |
URL | https://pubchem.ncbi.nlm.nih.gov | |
Description | Data deposited in or computed by PubChem | |
Canonical SMILES |
CCN(CC)C1=NC2=C(N=NN2C3=CC(=C(C=C31)OC)OC)S(=O)(=O)C4=CC=C(C=C4)Cl | |
Source | PubChem | |
URL | https://pubchem.ncbi.nlm.nih.gov | |
Description | Data deposited in or computed by PubChem | |
Molecular Formula |
C21H22ClN5O4S | |
Source | PubChem | |
URL | https://pubchem.ncbi.nlm.nih.gov | |
Description | Data deposited in or computed by PubChem | |
DSSTOX Substance ID |
DTXSID80659297 | |
Record name | 3-(4-Chlorobenzene-1-sulfonyl)-N,N-diethyl-7,8-dimethoxy[1,2,3]triazolo[1,5-a]quinazolin-5-amine | |
Source | EPA DSSTox | |
URL | https://comptox.epa.gov/dashboard/DTXSID80659297 | |
Description | DSSTox provides a high quality public chemistry resource for supporting improved predictive toxicology. | |
Molecular Weight |
475.9 g/mol | |
Source | PubChem | |
URL | https://pubchem.ncbi.nlm.nih.gov | |
Description | Data deposited in or computed by PubChem | |
CAS No. |
1200443-21-5 | |
Record name | 3-(4-Chlorobenzene-1-sulfonyl)-N,N-diethyl-7,8-dimethoxy[1,2,3]triazolo[1,5-a]quinazolin-5-amine | |
Source | EPA DSSTox | |
URL | https://comptox.epa.gov/dashboard/DTXSID80659297 | |
Description | DSSTox provides a high quality public chemistry resource for supporting improved predictive toxicology. | |
Retrosynthesis Analysis
AI-Powered Synthesis Planning: Our tool employs the Template_relevance Pistachio, Template_relevance Bkms_metabolic, Template_relevance Pistachio_ringbreaker, Template_relevance Reaxys, Template_relevance Reaxys_biocatalysis model, leveraging a vast database of chemical reactions to predict feasible synthetic routes.
One-Step Synthesis Focus: Specifically designed for one-step synthesis, it provides concise and direct routes for your target compounds, streamlining the synthesis process.
Accurate Predictions: Utilizing the extensive PISTACHIO, BKMS_METABOLIC, PISTACHIO_RINGBREAKER, REAXYS, REAXYS_BIOCATALYSIS database, our tool offers high-accuracy predictions, reflecting the latest in chemical research and data.
Strategy Settings
Precursor scoring | Relevance Heuristic |
---|---|
Min. plausibility | 0.01 |
Model | Template_relevance |
Template Set | Pistachio/Bkms_metabolic/Pistachio_ringbreaker/Reaxys/Reaxys_biocatalysis |
Top-N result to add to graph | 6 |
Feasible Synthetic Routes
試験管内研究製品の免責事項と情報
BenchChemで提示されるすべての記事および製品情報は、情報提供を目的としています。BenchChemで購入可能な製品は、生体外研究のために特別に設計されています。生体外研究は、ラテン語の "in glass" に由来し、生物体の外で行われる実験を指します。これらの製品は医薬品または薬として分類されておらず、FDAから任何の医療状態、病気、または疾患の予防、治療、または治癒のために承認されていません。これらの製品を人間または動物に体内に導入する形態は、法律により厳格に禁止されています。これらのガイドラインに従うことは、研究と実験において法的および倫理的な基準の遵守を確実にするために重要です。