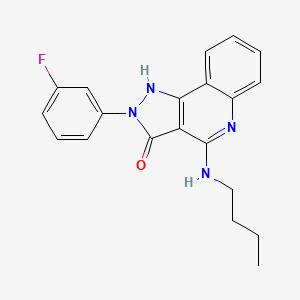
PQ-69
- 専門家チームからの見積もりを受け取るには、QUICK INQUIRYをクリックしてください。
- 品質商品を競争力のある価格で提供し、研究に集中できます。
説明
PQ 69は、逆アゴニスト活性を持つ新規で選択的なアデノシンA1受容体アンタゴニストです。 この化合物は有望な薬物動態学的特性を示しており、特に利尿薬および降圧薬としての潜在的な治療用途について研究されています .
科学的研究の応用
PQ 69 has a wide range of scientific research applications, including:
Chemistry: PQ 69 is used as a research tool to study the function of adenosine A1 receptors and their role in various physiological processes.
Biology: In biological research, PQ 69 is used to investigate the effects of adenosine A1 receptor antagonism on cellular signaling pathways.
Medicine: PQ 69 is being explored as a potential therapeutic agent for conditions such as hypertension and diuresis due to its ability to increase urine flow and sodium excretion
Industry: PQ 69 may have applications in the pharmaceutical industry for the development of new drugs targeting adenosine A1 receptors.
作用機序
PQ 69は、アデノシンA1受容体に選択的に結合して拮抗することによってその効果を発揮します。この受容体は、心拍数、腎機能、神経伝達物質の放出などの様々な生理学的プロセスに関与しています。 アデノシンA1受容体を阻害することにより、PQ 69は受容体の活性を阻害し、尿流量とナトリウム排泄量の増加につながります . 関与する分子標的と経路には、アデニル酸シクラーゼの阻害、これは環状AMPレベルの低下をもたらし、Gタンパク質を介したホスホリパーゼCとホスホリパーゼDの活性化が含まれます .
類似の化合物との比較
PQ 69は、アデノシンA1受容体アンタゴニストとしての高い選択性と効力においてユニークです。類似の化合物には、次のようなものがあります。
1,3-ジプロピル-8-シクロペンチルキサンチン: アデノシンA1受容体アンタゴニストですが、PQ 69よりも選択性と効力が低いです.
2-クロロ-N6-シクロペンチルアデノシン: 受容体機能を研究するために研究で使用されるアデノシンA1受容体アゴニスト.
PQ 69は、類似の化合物と比べて代謝安定性が向上し、末端排泄半減期が長いなど、良好な薬物動態学的特性を持つため、際立っています .
生化学分析
Biochemical Properties
PQ-69 plays a crucial role in biochemical reactions by selectively antagonizing the adenosine A1 receptor (A1AR) . In vitro binding assays have demonstrated that this compound has a Ki value of 0.96 nM for the cloned human A1 receptor, indicating its high affinity and selectivity . This compound exhibits 217-fold selectivity for A1AR over A2A receptors and more than 1,000-fold selectivity over A3 receptors . The interaction of this compound with A1AR inhibits the receptor’s activity, leading to a decrease in cyclic AMP levels and modulation of various downstream signaling pathways .
Cellular Effects
This compound exerts significant effects on various cell types and cellular processes. By antagonizing the adenosine A1 receptor, this compound influences cell signaling pathways, gene expression, and cellular metabolism . The inhibition of A1AR by this compound results in reduced activation of G-protein-coupled signaling pathways, leading to decreased cyclic AMP levels and altered cellular responses . Additionally, this compound has been shown to inhibit the contraction of isolated guinea pig tissues induced by A1AR agonists, further demonstrating its impact on cellular function .
Molecular Mechanism
The molecular mechanism of this compound involves its binding to the adenosine A1 receptor, where it acts as an antagonist with inverse agonist activity . This binding inhibits the receptor’s ability to activate G-proteins, leading to a decrease in cyclic AMP production and modulation of downstream signaling pathways . This compound’s inverse agonist activity also suggests that it can stabilize the inactive conformation of the A1AR, further reducing its basal activity . These interactions at the molecular level contribute to the compound’s overall pharmacological effects .
Temporal Effects in Laboratory Settings
In laboratory settings, the effects of this compound have been observed to change over time. This compound exhibits good metabolic stability in vitro, with a longer terminal elimination half-life compared to other adenosine receptor antagonists . This stability allows for sustained activity and prolonged effects in experimental settings . Long-term studies have shown that this compound maintains its efficacy in modulating A1AR activity, with no significant degradation observed over extended periods .
Dosage Effects in Animal Models
The effects of this compound vary with different dosages in animal models. Systemic administration of this compound at doses of 0.03, 0.3, and 3 mg/kg has been shown to increase urine flow and sodium excretion in normal rats . These findings suggest that this compound has dose-dependent diuretic effects, with higher doses resulting in more pronounced physiological responses .
Metabolic Pathways
This compound is involved in several metabolic pathways, primarily through its interaction with the adenosine A1 receptor . The inhibition of A1AR by this compound leads to decreased cyclic AMP levels and modulation of various downstream signaling pathways . Additionally, this compound’s metabolic stability and longer elimination half-life contribute to its sustained activity in vivo .
Transport and Distribution
Within cells and tissues, this compound is transported and distributed through interactions with specific transporters and binding proteins . These interactions facilitate the compound’s localization and accumulation in target tissues, allowing it to exert its pharmacological effects . The selective binding of this compound to the adenosine A1 receptor also plays a crucial role in its distribution and activity within the body .
Subcellular Localization
This compound’s subcellular localization is primarily determined by its interaction with the adenosine A1 receptor . The compound’s binding to A1AR directs it to specific cellular compartments where it can modulate receptor activity and downstream signaling pathways . Additionally, any post-translational modifications or targeting signals associated with this compound may further influence its subcellular localization and function .
準備方法
PQ 69は、4-アルキルアミノ置換および2-アリールピラゾロ[4,3-c]キノリン-3-オン誘導体を含む一連の化学反応によって合成されます . 合成経路は通常、以下の手順を含みます。
ピラゾロキノリンコアの形成: この手順では、適切な前駆体を制御された条件下で環化します。
置換反応: アルキルハライドやアリールボロン酸などの試薬を使用して、アルキルアミノ基とアリール基を置換反応によって導入します。
精製: 最終生成物は、再結晶化やクロマトグラフィーなどの技術を使用して精製され、純粋な形でPQ 69が得られます。
PQ 69の工業的生産方法は、これらの合成経路をスケールアップし、反応条件を最適化して、高収率と純度を確保することになるでしょう。
化学反応の分析
PQ 69は、次のような様々な種類の化学反応を起こします。
酸化: PQ 69は特定の条件下で酸化され、酸化された誘導体を形成します。
還元: 還元反応は、水素化ホウ素ナトリウムなどの還元剤を使用して、PQ 69を還元型に変換することができます。
置換: PQ 69は、アルキルハライドやアリールボロン酸などの試薬を使用して、官能基を他の基で置換する置換反応を起こすことができます。
これらの反応で使用される一般的な試薬と条件には、過酸化水素などの酸化剤、水素化ホウ素ナトリウムなどの還元剤、パラジウム炭素などの触媒があります。これらの反応から生成される主要な生成物は、官能基が修飾されたPQ 69の様々な誘導体です。
科学研究への応用
PQ 69は、次のような幅広い科学研究への応用を持っています。
化学: PQ 69は、アデノシンA1受容体の機能と、様々な生理学的プロセスにおけるその役割を研究するための研究ツールとして使用されます。
生物学: 生物学的研究では、PQ 69はアデノシンA1受容体拮抗薬の細胞シグナル伝達経路への影響を調べるために使用されます。
医学: PQ 69は、尿流量とナトリウム排泄量を増加させる能力により、高血圧や利尿などの疾患に対する潜在的な治療薬として研究されています
産業: PQ 69は、アデノシンA1受容体を標的とする新薬の開発における製薬業界での応用があるかもしれません。
類似化合物との比較
PQ 69 is unique in its high selectivity and potency as an adenosine A1 receptor antagonist. Similar compounds include:
1,3-dipropyl-8-cyclopentylxanthine: Another adenosine A1 receptor antagonist, but with lower selectivity and potency compared to PQ 69.
2-chloro-N6-cyclopentyl adenosine: An adenosine A1 receptor agonist used in research to study receptor function.
PQ 69 stands out due to its favorable pharmacokinetic properties, including better metabolic stability and longer terminal elimination half-life compared to similar compounds .
特性
IUPAC Name |
4-(butylamino)-2-(3-fluorophenyl)-1H-pyrazolo[4,3-c]quinolin-3-one |
Source
|
---|---|---|
Source | PubChem | |
URL | https://pubchem.ncbi.nlm.nih.gov | |
Description | Data deposited in or computed by PubChem | |
InChI |
InChI=1S/C20H19FN4O/c1-2-3-11-22-19-17-18(15-9-4-5-10-16(15)23-19)24-25(20(17)26)14-8-6-7-13(21)12-14/h4-10,12,24H,2-3,11H2,1H3,(H,22,23) |
Source
|
Source | PubChem | |
URL | https://pubchem.ncbi.nlm.nih.gov | |
Description | Data deposited in or computed by PubChem | |
InChI Key |
FJRYQRBEISYVBB-UHFFFAOYSA-N |
Source
|
Source | PubChem | |
URL | https://pubchem.ncbi.nlm.nih.gov | |
Description | Data deposited in or computed by PubChem | |
Canonical SMILES |
CCCCNC1=NC2=CC=CC=C2C3=C1C(=O)N(N3)C4=CC(=CC=C4)F |
Source
|
Source | PubChem | |
URL | https://pubchem.ncbi.nlm.nih.gov | |
Description | Data deposited in or computed by PubChem | |
Molecular Formula |
C20H19FN4O |
Source
|
Source | PubChem | |
URL | https://pubchem.ncbi.nlm.nih.gov | |
Description | Data deposited in or computed by PubChem | |
Molecular Weight |
350.4 g/mol |
Source
|
Source | PubChem | |
URL | https://pubchem.ncbi.nlm.nih.gov | |
Description | Data deposited in or computed by PubChem | |
Q1: What is the primary biological target of PQ-69?
A1: this compound is a selective antagonist of the adenosine A1 receptor and exhibits inverse agonist activity. [] This means it binds to the adenosine A1 receptor and blocks the actions of adenosine, potentially leading to downstream effects opposite to those caused by adenosine binding.
試験管内研究製品の免責事項と情報
BenchChemで提示されるすべての記事および製品情報は、情報提供を目的としています。BenchChemで購入可能な製品は、生体外研究のために特別に設計されています。生体外研究は、ラテン語の "in glass" に由来し、生物体の外で行われる実験を指します。これらの製品は医薬品または薬として分類されておらず、FDAから任何の医療状態、病気、または疾患の予防、治療、または治癒のために承認されていません。これらの製品を人間または動物に体内に導入する形態は、法律により厳格に禁止されています。これらのガイドラインに従うことは、研究と実験において法的および倫理的な基準の遵守を確実にするために重要です。