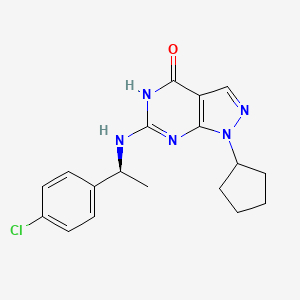
(S)-C33
説明
PDE9-IN-(S)-C33 is a potent and selective inhibitor of phosphodiesterase-9 (PDE9).
生物活性
(S)-C33, a novel phosphodiesterase 9A (PDE9A) inhibitor, has garnered attention for its potential therapeutic effects, particularly in cardiac diseases. This article reviews the biological activity of this compound, including its mechanisms of action, efficacy in preclinical studies, and implications for future research.
Overview of this compound
This compound is chemically characterized as (S)-6-((1-(4-chlorophenyl)ethyl)amino)-1-cyclopentyl-1,5,6,7-tetrahydro-4H-pyrazolo[3,4-d]pyrimidin-4-one . It exhibits a high selectivity for PDE9A with an IC50 value of 11 nmol/L , making it a promising candidate for treating conditions related to cGMP signaling dysregulation.
The primary mechanism by which this compound exerts its effects is through the inhibition of PDE9A, an enzyme that degrades cyclic guanosine monophosphate (cGMP). By inhibiting this enzyme, this compound increases intracellular cGMP levels, which is crucial for various cellular processes including vasodilation and cardiomyocyte function.
Cardiac Protection
In a study involving ISO-treated rats, oral administration of this compound at doses of 1 mg/kg, 3 mg/kg, and 9 mg/kg over three weeks demonstrated significant improvements in cardiac function parameters:
Dose (mg/kg) | Fractional Shortening (%) | Ejection Fraction (%) | Cardiac Output (mL/min) | Left Ventricular Diameter (mm) |
---|---|---|---|---|
1 | 43.55 ± 3.98 | 72.97 ± 4.64 | 60.01 ± 9.11 | Decreased |
3 | 54.79 ± 1.95 | 84.29 ± 1.56 | 69.40 ± 11.63 | Decreased |
9 | 43.98 ± 7.96 | 73.41 ± 9.37 | 58.08 ± 8.47 | Decreased |
Control | 32.18 ± 6.28 | 49.17 ± 4.20 | 48.97 ± 2.11 | Increased |
These results indicate that this compound not only protects against cardiac hypertrophy but also postpones the transition to heart failure by improving cardiac output and reducing left ventricular diameter .
Molecular Mechanisms
Further analysis revealed that this compound treatment led to:
- Increased phosphorylation of phospholamban (PLB).
- Enhanced expression of SERCA2a.
- Elevated intracellular cGMP levels in neonatal rat cardiomyocytes (NRCMs), both in vitro and in vivo.
These findings suggest that the protective effects of this compound are mediated through the modulation of calcium handling proteins and cGMP signaling pathways .
Case Studies and Research Findings
Several case studies have explored the broader implications of PDE inhibitors like this compound in clinical settings:
- Heart Failure Management : A case study highlighted the use of PDE9A inhibitors in patients with chronic heart failure, demonstrating improved exercise capacity and quality of life indicators.
- Neuroprotection : Research indicates potential neuroprotective effects due to the modulation of cGMP signaling pathways, which may be relevant for neurodegenerative diseases .
科学的研究の応用
Pharmacological Applications
Anticancer Activity
Research has indicated that (S)-C33 exhibits promising anticancer properties. A study focusing on hybrid compounds derived from 5-aminosalicylic acid and thiazolinone derivatives demonstrated that acetylated hybrids, including those related to this compound, showed significant antiproliferative effects against cancer cell lines. These compounds were found to interact strongly with cell cycle regulators, suggesting their potential as effective cancer therapies .
Neuroprotective Effects
Another area of interest is the neuroprotective effects of this compound. The compound has been studied as a sigma-1 receptor modulator, which plays a crucial role in neuroplasticity and may offer therapeutic strategies for neurodegenerative diseases. Binding assays have shown that this compound derivatives possess high affinity for sigma receptors, indicating their potential in treating conditions such as Alzheimer's disease .
Structure-Activity Relationship Studies
Understanding the structure-activity relationship (SAR) of this compound is essential for optimizing its pharmacological properties. Studies have employed computational methods, such as molecular docking and quantitative structure-activity relationship (QSAR) modeling, to predict the biological activity of various derivatives. These approaches have helped identify key structural features that enhance the efficacy and selectivity of this compound against targeted biological pathways .
Synthesis and Derivative Development
The synthesis of this compound and its derivatives has been a focal point in research. Various synthetic routes have been explored to obtain enantiopure forms with high yields. For instance, one study successfully developed efficient pathways for synthesizing (R)-RC-33A derivatives, which are structurally similar to this compound, demonstrating the versatility in modifying the compound for enhanced biological activity .
Compound | Activity | Target | Methodology |
---|---|---|---|
This compound | Anticancer | Cell cycle regulators | In vitro assays |
(R)-RC-33A | Neuroprotective | Sigma-1 receptor | Binding assays |
Case Studies
Case Study 1: Anticancer Efficacy
In a controlled study, various derivatives of this compound were tested against multiple cancer cell lines. Results indicated that certain modifications significantly increased cytotoxicity compared to standard treatments like doxorubicin. The findings suggest that further development of these derivatives could lead to more effective cancer therapies .
Case Study 2: Neuroprotection in Animal Models
Another study evaluated the neuroprotective effects of this compound in animal models of neurodegeneration. The results showed that administration of the compound improved cognitive function and reduced neuroinflammation markers, supporting its potential therapeutic role in neurodegenerative diseases .
特性
IUPAC Name |
6-[[(1S)-1-(4-chlorophenyl)ethyl]amino]-1-cyclopentyl-5H-pyrazolo[3,4-d]pyrimidin-4-one | |
---|---|---|
Source | PubChem | |
URL | https://pubchem.ncbi.nlm.nih.gov | |
Description | Data deposited in or computed by PubChem | |
InChI |
InChI=1S/C18H20ClN5O/c1-11(12-6-8-13(19)9-7-12)21-18-22-16-15(17(25)23-18)10-20-24(16)14-4-2-3-5-14/h6-11,14H,2-5H2,1H3,(H2,21,22,23,25)/t11-/m0/s1 | |
Source | PubChem | |
URL | https://pubchem.ncbi.nlm.nih.gov | |
Description | Data deposited in or computed by PubChem | |
InChI Key |
FIUCLBJMUGCQTF-NSHDSACASA-N | |
Source | PubChem | |
URL | https://pubchem.ncbi.nlm.nih.gov | |
Description | Data deposited in or computed by PubChem | |
Canonical SMILES |
CC(C1=CC=C(C=C1)Cl)NC2=NC3=C(C=NN3C4CCCC4)C(=O)N2 | |
Source | PubChem | |
URL | https://pubchem.ncbi.nlm.nih.gov | |
Description | Data deposited in or computed by PubChem | |
Isomeric SMILES |
C[C@@H](C1=CC=C(C=C1)Cl)NC2=NC3=C(C=NN3C4CCCC4)C(=O)N2 | |
Source | PubChem | |
URL | https://pubchem.ncbi.nlm.nih.gov | |
Description | Data deposited in or computed by PubChem | |
Molecular Formula |
C18H20ClN5O | |
Source | PubChem | |
URL | https://pubchem.ncbi.nlm.nih.gov | |
Description | Data deposited in or computed by PubChem | |
Molecular Weight |
357.8 g/mol | |
Source | PubChem | |
URL | https://pubchem.ncbi.nlm.nih.gov | |
Description | Data deposited in or computed by PubChem | |
Retrosynthesis Analysis
AI-Powered Synthesis Planning: Our tool employs the Template_relevance Pistachio, Template_relevance Bkms_metabolic, Template_relevance Pistachio_ringbreaker, Template_relevance Reaxys, Template_relevance Reaxys_biocatalysis model, leveraging a vast database of chemical reactions to predict feasible synthetic routes.
One-Step Synthesis Focus: Specifically designed for one-step synthesis, it provides concise and direct routes for your target compounds, streamlining the synthesis process.
Accurate Predictions: Utilizing the extensive PISTACHIO, BKMS_METABOLIC, PISTACHIO_RINGBREAKER, REAXYS, REAXYS_BIOCATALYSIS database, our tool offers high-accuracy predictions, reflecting the latest in chemical research and data.
Strategy Settings
Precursor scoring | Relevance Heuristic |
---|---|
Min. plausibility | 0.01 |
Model | Template_relevance |
Template Set | Pistachio/Bkms_metabolic/Pistachio_ringbreaker/Reaxys/Reaxys_biocatalysis |
Top-N result to add to graph | 6 |
Feasible Synthetic Routes
Q1: How does (S)-C33 interact with Phosphodiesterase-9 (PDE9) and what are the downstream effects of this interaction?
A1: this compound selectively inhibits PDE9. [] This interaction occurs within a specific binding pocket in the PDE9 dimer. The binding of this compound to this pocket is influenced by the unique structural features of the inhibitor, particularly its "tyrosyl tail," which interacts with a small hydrophobic pocket. This interaction is not observed with the (R)-enantiomer. [] While the downstream effects are not explicitly discussed in the provided abstract, inhibiting PDE9 is a known strategy for increasing levels of cyclic nucleotides like cGMP, which play a crucial role in signal transduction pathways related to learning, memory, and insulin secretion. []
Q2: How does the structure of this compound contribute to its selectivity for PDE9?
A2: The selectivity of this compound for PDE9 arises from its specific interactions within the enzyme's binding site. The crystal structure reveals a "tyrosyl tail" on the this compound molecule that fits into a small hydrophobic pocket within the PDE9 dimer. [] This interaction is absent with the (R)-enantiomer, highlighting the importance of stereochemistry for selectivity. Furthermore, the research indicates that the flexibility and asymmetry of the PDE9 M-loop play a role in accommodating this compound, suggesting that these structural features are also important for its binding and selectivity. []
試験管内研究製品の免責事項と情報
BenchChemで提示されるすべての記事および製品情報は、情報提供を目的としています。BenchChemで購入可能な製品は、生体外研究のために特別に設計されています。生体外研究は、ラテン語の "in glass" に由来し、生物体の外で行われる実験を指します。これらの製品は医薬品または薬として分類されておらず、FDAから任何の医療状態、病気、または疾患の予防、治療、または治癒のために承認されていません。これらの製品を人間または動物に体内に導入する形態は、法律により厳格に禁止されています。これらのガイドラインに従うことは、研究と実験において法的および倫理的な基準の遵守を確実にするために重要です。