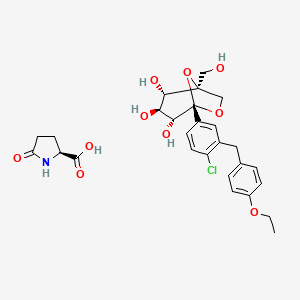
Ertugliflozin L-pyroglutamic acid
説明
Ertugliflozin L-pyroglutamic acid is a selective and orally active inhibitor of the sodium-dependent glucose cotransporter 2 (SGLT2). It is used primarily for the treatment of type 2 diabetes mellitus. This compound is known for its high potency, with an IC50 of 0.877 nM for h-SGLT2 . This compound is marketed under the brand name Steglatro and is also available in combination with other antidiabetic agents such as metformin and sitagliptin .
生化学分析
Biochemical Properties
Ertugliflozin L-pyroglutamic acid works by blocking glucose reabsorption from the glomerulus . It interacts with the SGLT2 enzyme, inhibiting its function . This interaction is highly selective, with this compound demonstrating over 2000-fold selectivity for SGLT2 inhibition relative to SGLT1 .
Cellular Effects
This compound influences cell function by reducing glucose reabsorption in the proximal tubules of the kidneys . This leads to increased urinary glucose excretion and reduced filtered glucose renal reabsorption .
準備方法
The preparation of Ertugliflozin L-pyroglutamic acid involves the synthesis of ertugliflozin followed by its conversion to the L-pyroglutamic acid co-crystal. The synthetic route typically involves the use of solvents such as alcohol or aqueous alcohol, and the process may include the use of excess L-pyroglutamic acid . An efficient process for the preparation of this compound has been developed, which is environmentally friendly and involves the preparation of substantially pure intermediates .
化学反応の分析
Ertugliflozin L-pyroglutamic acid undergoes various chemical reactions, including O-glucuronidation mediated by UGT1A9 and UGT2B7 to form pharmacologically inactive glucuronides . Additionally, about 12% of the compound undergoes CYP-mediated oxidative metabolism . The major products formed from these reactions are glucuronides and other metabolites found in plasma, feces, and urine .
科学的研究の応用
Ertugliflozin L-pyroglutamic acid has a wide range of scientific research applications. It is extensively studied for its efficacy in lowering A1C levels in adults with type 2 diabetes . The compound has been shown to be effective in multiple clinical trial settings and is used as an adjunct to diet and exercise to improve glycemic control .
作用機序
Ertugliflozin L-pyroglutamic acid works by inhibiting the sodium-dependent glucose cotransporter 2 (SGLT2), which is responsible for the reabsorption of glucose in the kidneys. By blocking this transporter, the compound increases urinary glucose excretion and reduces filtered glucose renal reabsorption . This mechanism of action leads to improved glycemic control in patients with type 2 diabetes . Additionally, the compound induces osmotic diuresis, which can lead to a mild reduction in blood pressure .
類似化合物との比較
Similar compounds in this class include dapagliflozin, canagliflozin, and empagliflozin . Compared to these compounds, Ertugliflozin L-pyroglutamic acid has a high potency and a favorable pharmacokinetic profile, with an absolute bioavailability of approximately 100% under fasted conditions . Additionally, it has been shown to be effective in combination with other antidiabetic agents such as metformin and sitagliptin .
特性
IUPAC Name |
(1S,2S,3S,4R,5S)-5-[4-chloro-3-[(4-ethoxyphenyl)methyl]phenyl]-1-(hydroxymethyl)-6,8-dioxabicyclo[3.2.1]octane-2,3,4-triol;(2S)-5-oxopyrrolidine-2-carboxylic acid | |
---|---|---|
Source | PubChem | |
URL | https://pubchem.ncbi.nlm.nih.gov | |
Description | Data deposited in or computed by PubChem | |
InChI |
InChI=1S/C22H25ClO7.C5H7NO3/c1-2-28-16-6-3-13(4-7-16)9-14-10-15(5-8-17(14)23)22-20(27)18(25)19(26)21(11-24,30-22)12-29-22;7-4-2-1-3(6-4)5(8)9/h3-8,10,18-20,24-27H,2,9,11-12H2,1H3;3H,1-2H2,(H,6,7)(H,8,9)/t18-,19-,20+,21-,22-;3-/m00/s1 | |
Source | PubChem | |
URL | https://pubchem.ncbi.nlm.nih.gov | |
Description | Data deposited in or computed by PubChem | |
InChI Key |
YHIUPZFKHZTLSH-LXYIGGQGSA-N | |
Source | PubChem | |
URL | https://pubchem.ncbi.nlm.nih.gov | |
Description | Data deposited in or computed by PubChem | |
Canonical SMILES |
CCOC1=CC=C(C=C1)CC2=C(C=CC(=C2)C34C(C(C(C(O3)(CO4)CO)O)O)O)Cl.C1CC(=O)NC1C(=O)O | |
Source | PubChem | |
URL | https://pubchem.ncbi.nlm.nih.gov | |
Description | Data deposited in or computed by PubChem | |
Isomeric SMILES |
CCOC1=CC=C(C=C1)CC2=C(C=CC(=C2)[C@@]34[C@@H]([C@H]([C@@H]([C@@](O3)(CO4)CO)O)O)O)Cl.C1CC(=O)N[C@@H]1C(=O)O | |
Source | PubChem | |
URL | https://pubchem.ncbi.nlm.nih.gov | |
Description | Data deposited in or computed by PubChem | |
Molecular Formula |
C27H32ClNO10 | |
Source | PubChem | |
URL | https://pubchem.ncbi.nlm.nih.gov | |
Description | Data deposited in or computed by PubChem | |
Molecular Weight |
566.0 g/mol | |
Source | PubChem | |
URL | https://pubchem.ncbi.nlm.nih.gov | |
Description | Data deposited in or computed by PubChem | |
CAS No. |
1210344-83-4 | |
Record name | Ertugliflozin pidolate | |
Source | ChemIDplus | |
URL | https://pubchem.ncbi.nlm.nih.gov/substance/?source=chemidplus&sourceid=1210344834 | |
Description | ChemIDplus is a free, web search system that provides access to the structure and nomenclature authority files used for the identification of chemical substances cited in National Library of Medicine (NLM) databases, including the TOXNET system. | |
Record name | Ertugliflozin | |
Source | European Chemicals Agency (ECHA) | |
URL | https://echa.europa.eu/information-on-chemicals | |
Description | The European Chemicals Agency (ECHA) is an agency of the European Union which is the driving force among regulatory authorities in implementing the EU's groundbreaking chemicals legislation for the benefit of human health and the environment as well as for innovation and competitiveness. | |
Explanation | Use of the information, documents and data from the ECHA website is subject to the terms and conditions of this Legal Notice, and subject to other binding limitations provided for under applicable law, the information, documents and data made available on the ECHA website may be reproduced, distributed and/or used, totally or in part, for non-commercial purposes provided that ECHA is acknowledged as the source: "Source: European Chemicals Agency, http://echa.europa.eu/". Such acknowledgement must be included in each copy of the material. ECHA permits and encourages organisations and individuals to create links to the ECHA website under the following cumulative conditions: Links can only be made to webpages that provide a link to the Legal Notice page. | |
Record name | Ertugliflozin pidolate | |
Source | FDA Global Substance Registration System (GSRS) | |
URL | https://gsrs.ncats.nih.gov/ginas/app/beta/substances/MLU731K321 | |
Description | The FDA Global Substance Registration System (GSRS) enables the efficient and accurate exchange of information on what substances are in regulated products. Instead of relying on names, which vary across regulatory domains, countries, and regions, the GSRS knowledge base makes it possible for substances to be defined by standardized, scientific descriptions. | |
Explanation | Unless otherwise noted, the contents of the FDA website (www.fda.gov), both text and graphics, are not copyrighted. They are in the public domain and may be republished, reprinted and otherwise used freely by anyone without the need to obtain permission from FDA. Credit to the U.S. Food and Drug Administration as the source is appreciated but not required. | |
Q1: How does Ertugliflozin L-pyroglutamic acid exert its therapeutic effect in diabetes?
A1: this compound is a selective inhibitor of sodium-glucose cotransporter 2 (SGLT2). [] SGLT2 is primarily located in the kidneys, where it plays a crucial role in reabsorbing glucose from the filtrate back into the bloodstream. By inhibiting SGLT2, this compound reduces glucose reabsorption in the kidneys, leading to increased urinary glucose excretion and lowering blood glucose levels in individuals with type 2 diabetes.
Q2: How does a standardized Public Assessment Report (PAR) contribute to better understanding of regulatory decisions regarding medications like this compound?
A3: A standardized PAR, utilizing tools like the Universal Methodology for Benefit-Risk Assessment (UMBRA) Benefit-Risk Template, can significantly improve transparency in regulatory decision-making. [] By including essential elements like regulatory history, a detailed effects table, and a clear record of strengths and uncertainties associated with each benefit and risk, stakeholders gain a more comprehensive understanding of the rationale behind regulatory approvals. [] This transparency is crucial for building trust and confidence in the regulatory process, especially for medications like this compound used in chronic conditions like type 2 diabetes.
試験管内研究製品の免責事項と情報
BenchChemで提示されるすべての記事および製品情報は、情報提供を目的としています。BenchChemで購入可能な製品は、生体外研究のために特別に設計されています。生体外研究は、ラテン語の "in glass" に由来し、生物体の外で行われる実験を指します。これらの製品は医薬品または薬として分類されておらず、FDAから任何の医療状態、病気、または疾患の予防、治療、または治癒のために承認されていません。これらの製品を人間または動物に体内に導入する形態は、法律により厳格に禁止されています。これらのガイドラインに従うことは、研究と実験において法的および倫理的な基準の遵守を確実にするために重要です。