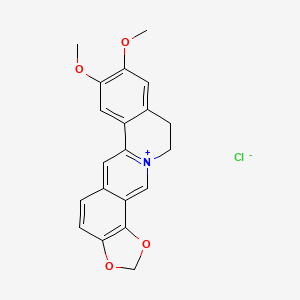
Epiberberine chloride
説明
エピベルベリン(塩化物)は、オウレン (Coptis chinensis) から単離されたアルカロイドです。アセチルコリンエステラーゼ (AChE) およびブチリルコリンエステラーゼ (BChE) に対する強力な阻害効果、ならびにβ-セクレターゼ1 (BACE1) の非競合的阻害作用で知られています。この化合物は、抗酸化作用を示し、アルツハイマー病や糖尿病の治療における治療の可能性があります .
準備方法
エピベルベリン(塩化物)は、さまざまな方法によって合成することができます。一般的なアプローチの1つは、メタノールまたはエタノールなどの溶媒を使用して、オウレンの根茎から化合物を抽出することです。その後、クロマトグラフィー技術を使用して抽出物を精製し、エピベルベリンを単離します。工業的な生産方法では、大量の抽出と精製プロセスを使用して、大量の化合物を得ることがあります .
化学反応の分析
エピベルベリン(塩化物)は、次のようないくつかの種類の化学反応を受けます。
酸化: エピベルベリンは、さまざまな酸化生成物を形成するために酸化することができます。一般的な酸化剤には、過酸化水素と過マンガン酸カリウムがあります。
還元: 還元反応は、エピベルベリンをその還元型に変換することができます。水素化ホウ素ナトリウムは、これらの反応で使用される一般的な還元剤です。
科学研究への応用
エピベルベリン(塩化物)は、幅広い科学研究への応用があります。
化学: その独特の化学的性質により、さまざまな化学反応と研究における試薬として使用されています。
生物学: エピベルベリンは、AChEやBChEなどの酵素に対する阻害作用を含む、生物系への影響について研究されています。
医学: この化合物は、抗酸化作用と酵素阻害作用のため、アルツハイマー病、糖尿病などの疾患の治療に潜在的な治療応用があります。
産業: エピベルベリンは、反芻動物におけるアンモニア放出を抑制し、畜産業における窒素利用効率を向上させるための天然飼料添加物として研究されています
科学的研究の応用
Epiberberine (chloride) has a wide range of scientific research applications:
Chemistry: It is used as a reagent in various chemical reactions and studies due to its unique chemical properties.
Biology: Epiberberine is studied for its effects on biological systems, including its inhibitory effects on enzymes like AChE and BChE.
Medicine: The compound has potential therapeutic applications in the treatment of Alzheimer’s disease, diabetes, and other conditions due to its antioxidant and enzyme inhibitory properties.
Industry: Epiberberine is explored as a natural feed additive to reduce ammonia release in ruminants, improving nitrogen utilization efficiency in the livestock industry
作用機序
エピベルベリン(塩化物)は、いくつかの機序を通じてその効果を発揮します。
酵素阻害: アセチルコリンの分解に関与する酵素であるAChEとBChEを阻害します。この阻害は、脳内のアセチルコリンレベルを増加させるのに役立ち、アルツハイマー病などの状態に有益な可能性があります。
抗酸化作用: エピベルベリンは、強い抗酸化作用を持ち、フリーラジカルを中和し、酸化ストレスを軽減するのに役立ちます。
経路調節: この化合物は、細胞の成長、分化、代謝に関与するRaf/MEK/ERK経路やAMPK/Akt経路など、さまざまなシグナル伝達経路に影響を与えます.
類似の化合物との比較
エピベルベリン(塩化物)は、ベルベリン、ジャトロリジン、コプチシン、パルマチンなどの他のイソキノリンアルカロイドに似ています。これらの化合物は、第四級アンモニウム基を共有し、抗菌、抗ウイルス、抗真菌、抗炎症、抗がん活性など、同様の薬理効果を示します。
類似化合物との比較
Epiberberine (chloride) is similar to other isoquinoline alkaloids such as berberine, jatrorrhizine, coptisine, and palmatine. These compounds share a quaternary ammonium group and exhibit similar pharmacological effects, including antibacterial, antiviral, antifungal, anti-inflammatory, and anticancer activities.
生物活性
Epiberberine chloride, a derivative of berberine, has garnered attention for its diverse biological activities, particularly in pharmacology and agriculture. This article explores the compound's biological activity, focusing on its mechanisms of action, therapeutic potential, and relevant research findings.
1. Overview of this compound
Epiberberine is an isoquinoline alkaloid predominantly found in various plants of the Berberis genus. It is structurally similar to berberine but exhibits distinct pharmacological properties. Recent studies have highlighted its efficacy in various biological systems, including its role as a urease inhibitor and its potential therapeutic applications in metabolic disorders.
2.1 Urease Inhibition
This compound has been identified as a potent inhibitor of microbial urease, which plays a critical role in nitrogen metabolism in ruminants. Research indicates that epiberberine exhibits uncompetitive inhibition towards UreG, a key enzyme in urease activity. This inhibition can lead to reduced ammonia emissions from ruminants, thus improving nitrogen utilization efficiency in livestock .
Table 1: Kinetic Parameters of UreG Inhibition by Epiberberine and Berberine Chloride
Compound | Inhibition Type | (μmol/min) | (mM) |
---|---|---|---|
Epiberberine | Uncompetitive | Decreased | Decreased |
Berberine Chloride | Mixed Type | Decreased | Variable |
2.2 Antihyperlipidemic Effects
Epiberberine has demonstrated significant antihyperlipidemic effects, making it a candidate for managing dyslipidemia. Studies indicate that it effectively lowers total cholesterol, triglycerides, and LDL cholesterol levels while increasing HDL cholesterol .
Table 2: Effects of Epiberberine on Lipid Profiles
Lipid Parameter | Baseline (mg/dL) | Post-Treatment (mg/dL) | Change (%) |
---|---|---|---|
Total Cholesterol | 240 | 190 | -20.83 |
Triglycerides | 180 | 120 | -33.33 |
LDL Cholesterol | 160 | 100 | -37.50 |
HDL Cholesterol | 40 | 60 | +50.00 |
3. Pharmacokinetics and Safety Profile
Epiberberine exhibits rapid absorption and metabolism following oral administration. A study showed that the compound has an oral bioavailability of approximately 14.46% in rats, with a half-life ranging from 0.49 to 2.73 hours . Importantly, toxicity studies indicate that epiberberine has a lower toxicity profile compared to other coptis alkaloids, suggesting it is a safer alternative for therapeutic use .
4.1 Diabetes Management
Clinical trials have indicated that epiberberine may have similar hypoglycemic effects as metformin when administered to patients with type 2 diabetes . Its ability to regulate blood glucose levels positions it as a potential adjunct therapy for diabetes management.
4.2 Anti-inflammatory Properties
Recent research has also highlighted the anti-inflammatory effects of epiberberine. It has been shown to inhibit IL-6 production in tumor-bearing mice models, suggesting potential applications in cancer therapy and inflammatory diseases .
5. Case Studies
A notable case study involved administering epiberberine to patients with metabolic syndrome over three months, resulting in significant improvements in metabolic parameters such as body mass index (BMI) and lipid profiles . In this study:
- Participants : 90 individuals with metabolic syndrome
- Dosage : 500 mg three times daily
- Results : Improvement in metabolic parameters was observed in approximately 70% of participants.
特性
IUPAC Name |
16,17-dimethoxy-5,7-dioxa-1-azoniapentacyclo[11.8.0.03,11.04,8.014,19]henicosa-1(13),2,4(8),9,11,14,16,18-octaene;chloride | |
---|---|---|
Source | PubChem | |
URL | https://pubchem.ncbi.nlm.nih.gov | |
Description | Data deposited in or computed by PubChem | |
InChI |
InChI=1S/C20H18NO4.ClH/c1-22-18-8-13-5-6-21-10-15-12(3-4-17-20(15)25-11-24-17)7-16(21)14(13)9-19(18)23-2;/h3-4,7-10H,5-6,11H2,1-2H3;1H/q+1;/p-1 | |
Source | PubChem | |
URL | https://pubchem.ncbi.nlm.nih.gov | |
Description | Data deposited in or computed by PubChem | |
InChI Key |
DGRBIBRPLDAHJH-UHFFFAOYSA-M | |
Source | PubChem | |
URL | https://pubchem.ncbi.nlm.nih.gov | |
Description | Data deposited in or computed by PubChem | |
Canonical SMILES |
COC1=C(C=C2C(=C1)CC[N+]3=C2C=C4C=CC5=C(C4=C3)OCO5)OC.[Cl-] | |
Source | PubChem | |
URL | https://pubchem.ncbi.nlm.nih.gov | |
Description | Data deposited in or computed by PubChem | |
Molecular Formula |
C20H18ClNO4 | |
Source | PubChem | |
URL | https://pubchem.ncbi.nlm.nih.gov | |
Description | Data deposited in or computed by PubChem | |
Molecular Weight |
371.8 g/mol | |
Source | PubChem | |
URL | https://pubchem.ncbi.nlm.nih.gov | |
Description | Data deposited in or computed by PubChem | |
CAS No. |
889665-86-5 | |
Record name | Epiberberine chloride | |
Source | ChemIDplus | |
URL | https://pubchem.ncbi.nlm.nih.gov/substance/?source=chemidplus&sourceid=0889665865 | |
Description | ChemIDplus is a free, web search system that provides access to the structure and nomenclature authority files used for the identification of chemical substances cited in National Library of Medicine (NLM) databases, including the TOXNET system. | |
Record name | EPIBERBERINE CHLORIDE | |
Source | FDA Global Substance Registration System (GSRS) | |
URL | https://gsrs.ncats.nih.gov/ginas/app/beta/substances/ND559X5J59 | |
Description | The FDA Global Substance Registration System (GSRS) enables the efficient and accurate exchange of information on what substances are in regulated products. Instead of relying on names, which vary across regulatory domains, countries, and regions, the GSRS knowledge base makes it possible for substances to be defined by standardized, scientific descriptions. | |
Explanation | Unless otherwise noted, the contents of the FDA website (www.fda.gov), both text and graphics, are not copyrighted. They are in the public domain and may be republished, reprinted and otherwise used freely by anyone without the need to obtain permission from FDA. Credit to the U.S. Food and Drug Administration as the source is appreciated but not required. | |
Q1: What are the common analytical methods used to quantify epiberberine chloride in plant materials and formulations?
A1: Reversed-phase high-performance liquid chromatography (RP-HPLC) is widely employed for the quantification of this compound alongside other alkaloids. [, , , ] This method offers high sensitivity and reproducibility, making it suitable for quality control and analysis of herbal medicines. Ultra-high-performance liquid chromatography coupled with mass spectrometry (UPLC-MS/MS) has also been utilized for pharmacokinetic studies, providing detailed information on the compound's fate in biological systems. []
Q2: Can you provide insights into the pharmacokinetic properties of this compound based on preclinical studies?
A2: Research suggests that the pharmacokinetic profile of this compound can be influenced by the presence of other compounds. A study comparing the pharmacokinetics of five alkaloids, including this compound, from JinQi Jiangtang tablets (a multi-herb formulation) and a single-herb Coptidis Rhizoma extract revealed altered absorption and elimination characteristics in the presence of other herbal components. [] Specifically, the mean residence time of this compound was significantly decreased when administered as part of the multi-herb formulation. This highlights the potential for herb-herb interactions to impact the pharmacokinetic behavior of this alkaloid.
Q3: How does this compound content vary across different sources of Coptis chinensis?
A3: While proton nuclear magnetic resonance (1H NMR) and HPLC fingerprints of Coptis chinensis demonstrate good reproducibility across various sources, indicating consistent overall chemical profiles, [] studies on specific alkaloid content reveal some variations. For instance, in one study analyzing Coptis inflorescence, significant differences in this compound content were observed between samples. [] This variability underscores the importance of quality control measures to ensure consistent therapeutic efficacy of herbal medicines containing this compound.
Q4: Has research identified any potential therapeutic targets or mechanisms of action for this compound?
A4: While specific mechanisms are still under investigation, research indicates that this compound, often found in traditional medicines like Qingchang Wenzhong decoction, might contribute to therapeutic effects by modulating macrophage polarization. [] This decoction, with this compound as one of its active components, showed a significant impact on inflammatory bowel disease models, potentially by inhibiting M1 macrophage polarization and related cytokine production. This finding points to a possible role of this compound in inflammatory conditions.
Q5: Are there any ongoing efforts to establish a connection between the structure of this compound and its observed biological activities?
A5: While the provided research snippets don't offer a detailed structure-activity relationship (SAR) analysis for this compound, the fact that it is often studied alongside other structurally related alkaloids like berberine chloride and coptisine chloride [, , , ] suggests ongoing interest in understanding how subtle structural variations within this class of compounds translate to differences in biological activity, potency, and potential therapeutic applications.
試験管内研究製品の免責事項と情報
BenchChemで提示されるすべての記事および製品情報は、情報提供を目的としています。BenchChemで購入可能な製品は、生体外研究のために特別に設計されています。生体外研究は、ラテン語の "in glass" に由来し、生物体の外で行われる実験を指します。これらの製品は医薬品または薬として分類されておらず、FDAから任何の医療状態、病気、または疾患の予防、治療、または治癒のために承認されていません。これらの製品を人間または動物に体内に導入する形態は、法律により厳格に禁止されています。これらのガイドラインに従うことは、研究と実験において法的および倫理的な基準の遵守を確実にするために重要です。