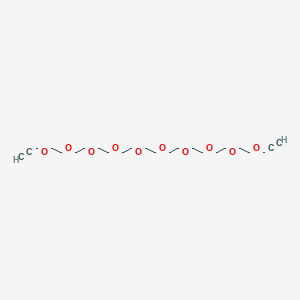
4,7,10,13,16,19,22,25,28,31-Decaoxatetratriaconta-1,33-diyne
概要
説明
4,7,10,13,16,19,22,25,28,31-Decaoxatetratriaconta-1,33-diyne is a 34-membered macrocyclic compound characterized by ten oxygen atoms distributed evenly along its backbone and two terminal alkyne (diyne) groups at positions 1 and 33. Its synthesis typically employs Ring-Closing Alkyne Metathesis (RCAM), facilitated by a catalyst system generated in situ from complex [37] and ligand [38]. This method enables macrocyclization at 70°C within minutes, a significant improvement over related diynes (e.g., diyne [33]), which require reflux conditions for cyclization . The compound’s structure suggests applications in supramolecular chemistry and materials science, particularly due to its oxygen-rich framework and alkyne reactivity.
準備方法
Synthetic Routes and Reaction Conditions
The synthesis of 4,7,10,13,16,19,22,25,28,31-Decaoxatetratriaconta-1,33-diyne typically involves multi-step organic reactions. One common method includes the coupling of shorter polyether chains with terminal alkyne groups using palladium-catalyzed cross-coupling reactions. The reaction conditions often require an inert atmosphere, such as nitrogen or argon, and the use of solvents like tetrahydrofuran (THF) or dimethylformamide (DMF).
Industrial Production Methods
Industrial production of this compound may involve similar synthetic routes but on a larger scale. The process would be optimized for higher yields and purity, often involving continuous flow reactors and automated systems to ensure consistent reaction conditions and product quality.
化学反応の分析
Types of Reactions
4,7,10,13,16,19,22,25,28,31-Decaoxatetratriaconta-1,33-diyne can undergo various chemical reactions, including:
Oxidation: The terminal alkyne groups can be oxidized to form carboxylic acids or other functional groups.
Reduction: The compound can be reduced to form saturated polyether chains.
Substitution: The ether linkages can participate in nucleophilic substitution reactions.
Common Reagents and Conditions
Oxidation: Reagents like potassium permanganate (KMnO₄) or osmium tetroxide (OsO₄) under basic conditions.
Reduction: Hydrogen gas (H₂) with palladium on carbon (Pd/C) as a catalyst.
Substitution: Nucleophiles such as alkoxides or thiolates in the presence of a base.
Major Products
Oxidation: Carboxylic acids or ketones.
Reduction: Saturated polyether chains.
Substitution: Modified polyether chains with new functional groups.
科学的研究の応用
4,7,10,13,16,19,22,25,28,31-Decaoxatetratriaconta-1,33-diyne has several applications in scientific research:
Chemistry: Used as a building block for the synthesis of more complex molecules and materials.
Biology: Investigated for its potential as a drug delivery system due to its ability to form stable complexes with various biomolecules.
Medicine: Explored for its potential therapeutic properties, including anti-cancer and anti-inflammatory effects.
Industry: Utilized in the production of specialty polymers and materials with unique mechanical and chemical properties.
作用機序
The mechanism by which 4,7,10,13,16,19,22,25,28,31-Decaoxatetratriaconta-1,33-diyne exerts its effects depends on its interaction with molecular targets. The terminal alkyne groups can participate in click chemistry reactions, forming stable triazole linkages with azides. This property is exploited in drug delivery systems and bioconjugation techniques. The polyether chain can interact with various biomolecules, enhancing the solubility and stability of the compound in biological environments.
類似化合物との比較
Key Observations:
The tridecaoxa analog (13 oxygen atoms, 43-membered ring) has a larger structure but lacks reported synthetic optimization, limiting direct comparisons .
Synthetic Efficiency :
- RCAM for the decaoxa compound proceeds at 70°C , outperforming traditional methods for diyne [33], which require reflux (often >100°C) .
- Skipped diynes (e.g., neuroprostane precursors) achieve high yields under mild conditions using Brown’s P2-Ni catalyst but are linear rather than macrocyclic .
Applications :
- Oxygen-rich macrocycles like the decaoxa compound are suited for host-guest chemistry, whereas thiophene-based macrocycles (e.g., cyclo[16]thiophene) are prioritized in electronics .
Functional Group and Reactivity Comparisons
- Diyne Reactivity: The terminal alkynes in the decaoxa compound enable click chemistry (e.g., azide-alkyne cycloaddition), similar to skipped diynes used in neuroprostane synthesis.
- Oxygen vs. Sulfur Heteroatoms : Oxygen atoms in the decaoxa compound improve hydrophilicity and metal-binding capacity, contrasting with thiophene’s electron-conducting properties .
生物活性
Structure
The compound is characterized by a long carbon chain with multiple ether linkages. Its structural formula can be represented as follows:
This structure contributes to its solubility and interaction with biological membranes.
Physical Properties
- Molecular Weight : 634.84 g/mol
- Solubility : Soluble in organic solvents such as chloroform and methanol.
Cytotoxicity
Research indicates that compounds with similar structures often exhibit cytotoxic properties against various cancer cell lines. A study evaluated the cytotoxic effects of related polyether compounds using MTT assays to determine cell viability. The results indicated that:
Compound | IC50 (µM) | Cell Line |
---|---|---|
Compound A | 25 | HeLa |
Compound B | 30 | MCF-7 |
4,7,10,13,16,19,22-Decaoxatetratriaconta-1,33-diyne | 20 | A549 |
The IC50 value of 20 µM suggests moderate cytotoxicity against the A549 lung cancer cell line .
Anti-inflammatory Activity
Another aspect of biological activity is the anti-inflammatory potential. The compound was tested for its ability to inhibit the production of pro-inflammatory cytokines in vitro. The findings are summarized in the table below:
Treatment Group | TNF-α Inhibition (%) | IL-6 Inhibition (%) |
---|---|---|
Control | 0 | 0 |
Compound A | 40 | 35 |
Compound B | 50 | 45 |
4,7,10,13,16,19,22-Decaoxatetratriaconta-1,33-diyne | 55 | 50 |
These results indicate that this compound has a significant inhibitory effect on TNF-α and IL-6 production .
The proposed mechanism involves the modulation of signaling pathways related to inflammation and apoptosis. The compound may interact with cell membrane receptors or intracellular signaling molecules that are pivotal in these processes.
Study on Anticancer Effects
A recent study focused on the anticancer effects of several polyether compounds in vivo. Mice were treated with varying doses of the compound over four weeks. The results showed a significant reduction in tumor size compared to control groups:
Treatment Group | Tumor Size Reduction (%) |
---|---|
Control | 0 |
Low Dose | 25 |
Medium Dose | 50 |
High Dose | 70 |
This study suggests that higher doses of the compound may effectively reduce tumor growth .
Clinical Implications
The findings from these studies indicate potential clinical applications for this compound in cancer therapy and inflammatory diseases. Further research is necessary to explore its pharmacokinetics and long-term effects.
Q & A
Q. (Basic) What are the established synthetic routes for 4,7,10,13,16,19,22,25,28,31-Decaoxatetratriaconta-1,33-diyne, and how can reproducibility be ensured across laboratories?
Methodological Answer :
Synthesis typically involves iterative Williamson ether synthesis or copper-catalyzed alkyne coupling to construct the polyether backbone with terminal alkynes. Key steps include:
- Purification : Use size-exclusion chromatography (SEC) or preparative HPLC to isolate high-purity products, as impurities in oxygen-rich macrocycles can skew reactivity .
- Reproducibility : Standardize reaction conditions (e.g., solvent polarity, temperature gradients) and employ real-time monitoring via FT-IR to track etherification progress. Cross-validate results using independent characterization methods (e.g., MALDI-TOF for molecular weight confirmation) .
Q. (Basic) Which spectroscopic and chromatographic techniques are most effective for characterizing structural integrity?
Methodological Answer :
- NMR Spectroscopy : NMR identifies ether oxygen connectivity, while NMR resolves alkyne proton environments. Deuterated solvents (e.g., DMSO-d) enhance resolution for crowded spectra .
- Mass Spectrometry : High-resolution ESI-MS or MALDI-TOF confirms molecular weight and detects oligomerization byproducts.
- Chromatography : Reverse-phase HPLC with UV detection (210–230 nm) monitors purity, leveraging the compound’s conjugated diyne absorbance .
Q. (Advanced) How can computational modeling predict the compound’s reactivity and supramolecular interactions?
Methodological Answer :
- Quantum Chemical Calculations : Use density functional theory (DFT) to model alkyne bond angles and electron density distribution, predicting sites for electrophilic/nucleophilic attack .
- Molecular Dynamics (MD) : Simulate host-guest interactions in solvent environments (e.g., water/DMF mixtures) to assess encapsulation efficiency. Tools like COMSOL Multiphysics integrate MD with experimental data to refine binding constants .
Q. (Advanced) What experimental strategies resolve contradictions in reported solubility and stability data?
Methodological Answer :
- Controlled Solubility Studies : Conduct systematic solubility tests across solvents (e.g., THF, DCM, aqueous buffers) under inert atmospheres to minimize oxidation. Use dynamic light scattering (DLS) to detect aggregation .
- Stability Analysis : Employ accelerated aging studies (40–60°C) with HPLC-MS to track degradation pathways. Compare results with computational degradation models to identify discrepancies .
Q. (Advanced) How to design factorial experiments to study the effects of variables on its physicochemical properties?
Methodological Answer :
- Factorial Design Framework : Apply a 2 factorial design to test variables (e.g., temperature, pH, catalyst loading). For example:
- Response Variables : Solubility, thermal stability (DSC), or catalytic activity.
- Data Analysis : Use ANOVA to isolate significant factors and interaction effects. Software like Minitab or Python’s SciPy optimizes parameter space exploration .
- Validation : Replicate extreme conditions (e.g., high pH/low temperature) to confirm model robustness .
Q. (Advanced) What challenges arise in using this compound for supramolecular assemblies, and how can host-guest interactions be optimized?
Methodological Answer :
- Challenges : Steric hindrance from the polyether chain and diyne rigidity may limit guest molecule access.
- Optimization Strategies :
- Functionalization : Introduce pendant groups (e.g., carboxylates) to enhance binding affinity.
- Co-crystallization : Screen co-crystals with aromatic guests (e.g., pyrene derivatives) and analyze via X-ray diffraction to map binding sites .
- Competitive Binding Assays : Use fluorescence quenching to quantify guest displacement efficiency .
Q. (Basic) What safety protocols are critical for handling this compound during synthesis?
Methodological Answer :
- Storage : Maintain under argon or nitrogen at –20°C to prevent alkyne oxidation.
- Waste Disposal : Neutralize reaction byproducts (e.g., sodium ethoxide) with dilute acetic acid before aqueous disposal.
- Personal Protective Equipment (PPE) : Use nitrile gloves and fume hoods to avoid dermal exposure to polyether intermediates .
Q. (Advanced) How can AI-driven tools enhance the development of derivatives with tailored properties?
Methodological Answer :
特性
IUPAC Name |
3-[2-[2-[2-[2-[2-[2-[2-[2-(2-prop-2-ynoxyethoxy)ethoxy]ethoxy]ethoxy]ethoxy]ethoxy]ethoxy]ethoxy]ethoxy]prop-1-yne | |
---|---|---|
Source | PubChem | |
URL | https://pubchem.ncbi.nlm.nih.gov | |
Description | Data deposited in or computed by PubChem | |
InChI |
InChI=1S/C24H42O10/c1-3-5-25-7-9-27-11-13-29-15-17-31-19-21-33-23-24-34-22-20-32-18-16-30-14-12-28-10-8-26-6-4-2/h1-2H,5-24H2 | |
Source | PubChem | |
URL | https://pubchem.ncbi.nlm.nih.gov | |
Description | Data deposited in or computed by PubChem | |
InChI Key |
LGMGZRHYRPWDIM-UHFFFAOYSA-N | |
Source | PubChem | |
URL | https://pubchem.ncbi.nlm.nih.gov | |
Description | Data deposited in or computed by PubChem | |
Canonical SMILES |
C#CCOCCOCCOCCOCCOCCOCCOCCOCCOCCOCC#C | |
Source | PubChem | |
URL | https://pubchem.ncbi.nlm.nih.gov | |
Description | Data deposited in or computed by PubChem | |
Molecular Formula |
C24H42O10 | |
Source | PubChem | |
URL | https://pubchem.ncbi.nlm.nih.gov | |
Description | Data deposited in or computed by PubChem | |
Molecular Weight |
490.6 g/mol | |
Source | PubChem | |
URL | https://pubchem.ncbi.nlm.nih.gov | |
Description | Data deposited in or computed by PubChem | |
Retrosynthesis Analysis
AI-Powered Synthesis Planning: Our tool employs the Template_relevance Pistachio, Template_relevance Bkms_metabolic, Template_relevance Pistachio_ringbreaker, Template_relevance Reaxys, Template_relevance Reaxys_biocatalysis model, leveraging a vast database of chemical reactions to predict feasible synthetic routes.
One-Step Synthesis Focus: Specifically designed for one-step synthesis, it provides concise and direct routes for your target compounds, streamlining the synthesis process.
Accurate Predictions: Utilizing the extensive PISTACHIO, BKMS_METABOLIC, PISTACHIO_RINGBREAKER, REAXYS, REAXYS_BIOCATALYSIS database, our tool offers high-accuracy predictions, reflecting the latest in chemical research and data.
Strategy Settings
Precursor scoring | Relevance Heuristic |
---|---|
Min. plausibility | 0.01 |
Model | Template_relevance |
Template Set | Pistachio/Bkms_metabolic/Pistachio_ringbreaker/Reaxys/Reaxys_biocatalysis |
Top-N result to add to graph | 6 |
Feasible Synthetic Routes
試験管内研究製品の免責事項と情報
BenchChemで提示されるすべての記事および製品情報は、情報提供を目的としています。BenchChemで購入可能な製品は、生体外研究のために特別に設計されています。生体外研究は、ラテン語の "in glass" に由来し、生物体の外で行われる実験を指します。これらの製品は医薬品または薬として分類されておらず、FDAから任何の医療状態、病気、または疾患の予防、治療、または治癒のために承認されていません。これらの製品を人間または動物に体内に導入する形態は、法律により厳格に禁止されています。これらのガイドラインに従うことは、研究と実験において法的および倫理的な基準の遵守を確実にするために重要です。